EFFECT OF GHRELIN ON THE APOPTOSIS OF VARIOUS CELLS.
A CRITICAL REVIEW
Jagiellonian University in Cracow, Cracow, Poland
INTRODUCTION
Apoptosis, or programmed cell death, is a genetically controlled process where cells die in response to environmental or developmental cues. Regulation of apoptosis is critical for normal development and tissue homeostasis, and disruption of this process can have severe consequences (1). This programmed cell death plays a critical role in physiological functions such as cell deletion, development, differentiation, balancing cell number in continuously renewing tissue and immune system development. The process of apoptosis is controlled by a diverse range of cell signals, which may originate either extracellularly (extrinsic inducers) or intracellularly (intrinsic inducers). Extracellular signals include hormones, growth factors and cytokines that must either cross the plasma membrane or transduce to effect a response. These signals may positively or negatively affect apoptosis. In recent years, the role of ghrelin on cell apoptosis has been documented, although conflicting results in different cell types have been reported.
BIOLOGY OF GHRELIN: STRUCTURE AND FUNCTION
Ghrelin was isolated from the rat stomach in 1999 and was identified as a ligand for the growth hormone secretagogue receptor (GHSR) (2). The human GHRL gene, which is built from 4 coding and 2 noncoding exons as well as 4 introns, is mapped at chromosome 3p25.3 (3). The sequence is conserved in mammals including humans, rats, mice, rhesus monkeys, Mongolian gerbils, cows, pigs, sheep and dogs. Moreover, at the NH2 terminal, the 10 amino acids sequence is identical in these species (2, 4). Pre-proghrelin is a 117 amino acid precursor of ghrelin and from this, the 94 amino acids proghrelin is formed after cleavage. Finally, after further processing, the ghrelin protein is made, which contains 28 amino acids and an N-octanoyl group on the serine in position 3 (4). In the organism, ghrelin occurs in two forms: acylated and desacylated. The desacylated ghrelin is more abundant in blood (90%) than acylated (10%) (5). The gene encoding growth hormone secretagogue receptor (GHSR) is mapped at chromosome 3q26.31 and yields two variants, GHSR1a (functional ghrelin receptor) and GHSR1b (inactive, but it seems to modulate GHSR1a receptor activity) (6).
Ghrelin is expressed in the many tissues, including X/A-like cells of humans and rats and, in order of decreasing expression, duodenum, jejunum, ileum and colon. Ghrelin is also expressed in different organs such as hypothalamus, pancreas and ovaries, as well as interstitial cells of rats (4). In human plasma, the total ghrelin concentration is around 100 – 150 fmol/ml (7). The most important factor in regulating ghrelin expression is feeding (8). Plasma ghrelin concentration increases when fasting but decreases after food intake. Moreover, the concentration of ghrelin in patients with simple obesity is lower, and anorexia nervosa higher, compared to healthy individuals with normal body weight (9). GHSR is expressed in liver, kidney, placenta, T cells, myocardium, gastrointestinal tract and central nervous system components such as hypothalamus, pituitary, brain and stem (8).
Ghrelin is a hormone with different physiological functions (Fig. 1), is involved in growth hormone (GH)-releasing activity and stimulates the release of GH in humans at 1 µg/kg concentration. Stimulatory effect of ghrelin is also observed in prolactin release (10). Intravenous and subcutaneous injections of ghrelin increase food intake, while peripherally injected ghrelin stimulates hypothalamic neurons and food intake in rats (11). Furthermore, ghrelin administered intravenously at concentrations 0.8 to 20 µg/kg increases gastric motility and acid secretion in rats, and accelerates gastric emptying in preparation for re-ingestion (12, 13). Intravenous injection of ghrelin at 10 µg/kg in healthy humans decreases mean arterial pressure without changing the heart rate (14). Ghrelin also affects reproduction by suppressing luteinizing hormone (LH) secretions in rats, rhesus monkeys, sheep and humans (15). Study of Rak et al. (16) showed that ghrelin at doses 100 – 1000 pg/ml increases estradiol (E2) secretion and aromatase expression in in vitro cultures of pre-pubertal pig ovarian follicles. In bovine oocyte maturation in vitro experiment, ghrelin at dose 60 pM has a negative effect on cumulus viability and pronuclear formation (17). In pancreas of rats, ghrelin at doses 10 and 20 nM/kg plays an anti-inflammatory role by inhibiting the production of pro-inflammatory cytokines (18).
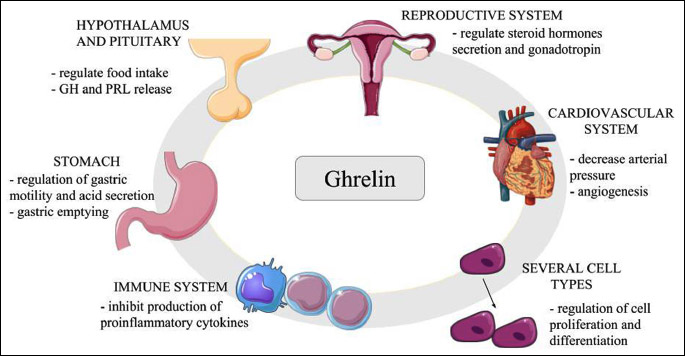
Abbreviations: GH, growth hormone; PRL, prolactin.
Ghrelin acts using different kinase pathways. For example, in human umbilical vein endothelial cells (HUVEC), ghrelin at doses 10–9 to 10–7 M stimulates endothelial cell angiogenesis and migration through the mitogen activated (MEK-ERK) signalling pathway. Contrastingly, inhibition of the ERK pathway cancels this effect (19). Ghrelin can also promote proliferation of adenocarcinomic, human alveolar basal epithelial cells (A549) via GHSR-dependent phosphatidylinositide 3-kinases/protein kinase B/mammalian target of rapamycin kinase/ribosomal protein S6 kinase beta-1 (PI3K/Akt/mTOR/P70S6K) and ERK signalling pathways. In contrast, inhibitors of PI3K, ERK and mTOR can block ghrelin-induced A549 cell proliferation (20). Lastly, in mesenchymal stem cells, ghrelin at 600 ng/ml concentration has been shown to impact cell differentiation through the ERK1/2 pathway (21).
CHARACTERISTICS OF APOPTOSIS
Apoptosis is a process which occurs normally during development, and also acts as a defence mechanism when the cells are damaged or as a response on immune reactions (22). Apoptosis was first named in 1972 by Kerr as a-po-toe-sis (23). During apoptosis, a number of morphological changes occur. For example, in early process of apoptosis, it is possible to observe cell shrinkage and pyknosis, where the cells become smaller, organelles tightly packed, cytoplasm denser and chromatin more condensed (23). Plasma membrane blebbing also occurs followed by karyorrhexis and separation of cell fragments into apoptotic bodies, in a process called “budding”. Apoptotic bodies contain a cytoplasm with organelles but with or without nuclear fragments. Moreover, organelle integrity is maintained. Apoptotic bodies are then phagocytosed and depredated in phagolysosome. All of these occur without inflammation (24, 25).
There are two distinct apoptotic pathways: extrinsic or death receptor pathway and intrinsic or mitochondrial pathway. These pathways can be linked (Fig. 2) (26). Both of them activate caspases, cysteine aspartyl proteases, and lead to mitochondrial membrane permeabilisation, chromatin condensation and DNA fragmentation, which ultimately end in cell destruction (27). Caspases can be categorised into initiators (caspase-2, -8, -9 and -10), effectors or executioners (caspase-3, -6 and -7) and inflammatory (caspase-1, -4 , -5, -11, -12) (28). One caspase can activate other caspases in a proteolytic cascade, leading to rapid cell death.
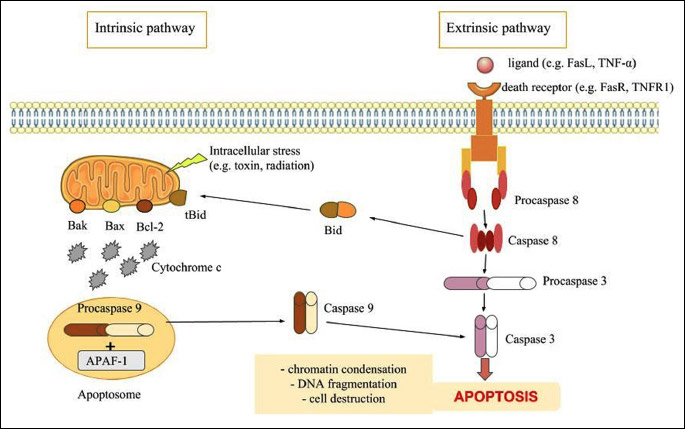
Abbreviations: APAF-1, apoptotic protease activating factor 1; Bcl-2, B-cell lymphoma 2; TNF-α, tumor necrosis factor alpha; TNFR1, tumor necrosis factor receptor 1.
In extrinsic signalling pathways, there occurs transmembrane receptor-mediated interactions caused by death receptors, which include members of the tumour necrosis factor (TNF) receptor gene superfamily (29). These receptors have cysteine-rich extracellular domains and 80 amino acids domain, which play a role in transmitting the death signal from the cell surface into the intracellular signalling pathways, called the “death domain” (30). The ligands for these receptors include FasL/FasR, tumour necrosis factor receptor 1 (TNF-α/TNFR1), Apo3L/DR3, Apo2L/DR4 and Apo2L/DR5 (30-34). After binding their ligand, the receptors start to oligomerise followed by the recruitment of an adaptor protein to the death domain. The adaptor protein then binds to caspases, which act as the effector protein (35).
The intrinsic signalling pathways are caused by non-receptor-mediated stimuli that produce intracellular signals that act directly on targets within the cell. In other words, they are mitochondrial-initiated events and can act positively (toxin, radiation) or negatively, for example, in the absence of growth factors, hormones or cytokines. This stimulation causes changes in the inner mitochondrial membrane, opening of the mitochondrial permeability transition (MPT) pore, loss of the mitochondrial transmembrane potential and release of pro-apoptotic proteins into the cytosol from the intermembrane space (36).
Some molecules participate in apoptosis regulation, such as members of the polypeptides homologous to B-cell lymphoma 2 (Bcl-2) including Bax, B-cell lymphoma-extra small (Bcl-xs) and bak, which promote apoptosis, as well as Bcl-2 and B-cell lymphoma-extra large (Bcl-xL), which suppress apoptosis (37). Apoptosis can also be regulated by cytosolic concentration and intracellular compartmentalisation of calcium ions (Ca2+). In thymocytes, treatment with thapsigargin, an inhibitor of the endoplasmic reticulum Ca2+ ATPase, can have pro-apoptotic effect (38). Moreover, overexpression of protein kinase C subtype α in breast cancer MCF-7 cell line can lead to selective sensitivity of a multidrug-resistant subclone, through phorbol ester-induced apoptosis (39). Cyclic adenosine monophosphate (cAMP)-induced apoptosis includes activation of cAMP-dependent protein kinase A (PKA) in thymocytes (40). However, cAMP can block apoptosis in neurons (41). Protein tyrosine kinases (PTKs) play important roles in suppressing apoptosis, while their antagonists can induce apoptosis in 32D cl3(G), a bone marrow mouse cells (42), and inhibit many survival factors in hematopoietic cells such as interleukin 2 (IL-2) and interleukin 3 (IL-3) (43).
EFFECT OF GHRELIN ON THE APOPTOSIS OF VARIOUS CELLS
Apoptosis plays a critical role in physiological processes such as cell deletion, organ development, differentiation, proliferation, balancing cell number in continuously renewing tissue and immune system development. It is also well known fact that dysfunction or dysregulation of apoptosis leads to pathology conditions. Several published study described controversial, stimulatory, inhibitory or no action of ghrelin on cell apoptosis in in vitro and in vivo experiments. Ghrelin inhibits apoptosis in many different cells like ovarian, cardiomyocytes, mucosa, neuronal, endothelial and cancer (Table 1), promote apoptosis in oocyte, colon adenocarcinoma, lung, adrenocortical tumor cells and rheumatoid arthritis fibroblast-like synoviocyte (MH7A) (Table 2), or have no effects on apoptosis in HUVEC, human normal adrenocortical cells, in rat osteoblasts, thymocytes or zona glomerulosa cells (Table 3).
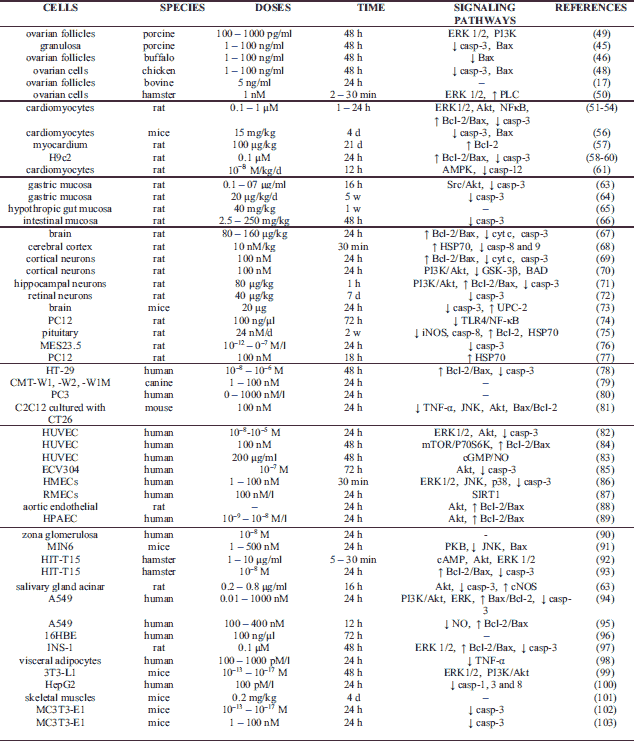
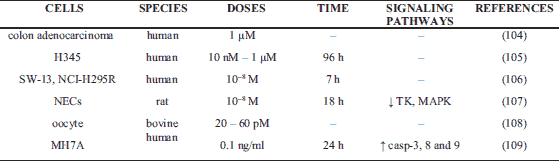
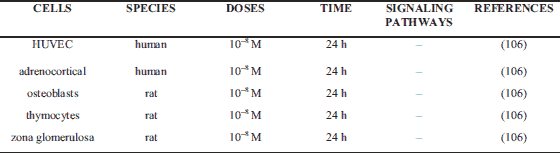
Anti-apoptotic effect of ghrelin in ovary
In the ovaries, apoptosis has been implicated in a spectrum of processes associated with normal ovarian development and function, including prenatal germ cell attrition, postnatal follicular atresia, ovulation and luteolysis. Ghrelin at doses 100 – 1000 pg/ml decreases caspase-3 activity and the rates of DNA fragmentation in the pig ovarian cells (16, 45). Authors observed that stimulatory action of ghrelin on E2 secretion is due to its anti-apoptotic effect in the ovarian follicles (16), suggests a direct interference of oestrogens with apoptotic processes. Recently, E2 has been found to prevent caspase-6-mediated neuronal cell death, possibly by introducing a caspase inhibitory factor through a receptor-mediated non-genomic pathway (44). Another example in support of this hypothesis is the stimulation of the expression of anti-apoptotic proteins, such as Bcl-2 or Bcl-xl. In buffalo ovarian follicles, ghrelin promotes cell proliferation, as measured by PCNA level, and anti-apoptosis, as measured by Bax level (46). As demonstrated by Sirotkin et al. (47), the ghrelin analogue, ghrelin 1-18, at 1, 10 and 100 ng/ml decreases the expression of apoptotic markers in chicken ovarian follicular fragments. Interestingly, the net effects of ghrelin on apoptosis appear to be dependent on the different cellular compartments of chicken ovary, as ghrelin-induced changes in apoptotic indexes in isolated granulosa cells (reduction in proportion of cells containing Bax, caspase and Bcl-2, as well as in TUNEL-positive cells) are not similar to those induced in cultures of whole chicken follicular wall fragments (increase in accumulation of Bax and p53). Additionally, observations made by Sirotkin and Grossmann (48) indicate that different parts of ghrelin molecule could be responsible for varying ghrelin effects, although the major ghrelin action is probably associated with its 1-18 residues. Ghrelin mediates cell apoptosis in the ovarian cells through activation of ERK1/2 and PI3K kinases (49). Additionally, Sirotkin et al. (47) showed that MAPK, tyrosine kinases and cyclic-dependent protein kinases could also be regulators of avian ovarian secretion and intracellular mediators of ghrelin action in the ovary. Mousseaux et al. (50) suggest that ghrelin, through GHSR-1a, activates ERK1/2 in Chinese hamster ovary cells. Additionally, they also indicate that phospholipase C (PLC) is involved in GHSR-1a-mediated ERK1/2 activation.
Anti-apoptotic effect of ghrelin in cardiomyocytes
Ghrelin is expressed in mammalian hearts and has protective effect on heart cells, but the mechanism of this action is still unknown and independent of GH release. In primary cultures of adult rat H9c2 cardiomyocyte cells, ghrelin at doses 0.1, 0.5 and 1.0 µM inhibits apoptosis by activating ERK1/2 and Akt kinases pathways (51). Effect of Akt kinase activation on apoptosis suppression is shown also in rat primary cardiomyocytes, where ghrelin improves integrity of cardiomyocytes and decreases shrinkage (52). In the apoptosis induced by high glucose and sodium palmitate, ghrelin has protective effect on cardiomyocytes of adult rats, also through activation of the PI3K-Akt signalling pathway. The other signalling pathway activated by ghrelin in this model is nuclear factor kappa B (NFκB), which increases the transcription of genes that participates in cells survival, Bcl-2 and Bcl-xL (53). Wang et al. (54) confirm on neonatal rat cardiomyocytes that ghrelin decreases apoptosis, Bax and caspase-3, but increases Bcl-2 expression by activation of the Akt pathways. During doxorubicin-induced cardiomyopathy in cultures of rat primary cardiomyocytes, ghrelin increases TNF-α and subsequently anti-oxidative and anti-apoptotic effects (55). In mice model of doxorubicin-induced cardiomyopathy, ghrelin is confirmed to have anti-apoptotic effect by decreasing Bax and caspase-3 activities (56). Also in rats, 21 days after myocardial infarction, ghrelin decreases the same pro-apoptotic factors while increasing anti-apoptotic Bcl-2 (57). Angiotensin II (Ang II) production is increased in heart failure, which can induce apoptosis of cardiomyocytes. Ghrelin in H9c2 cardiomyocytes regulates the Ang II-induced imbalance of Bax and Bcl-2 expression, as well as reduces Ang II-induced caspase-3 expression (58, 59). In rat model, ghrelin in in vivo and in vitro studies inhibits caspase-3 activity (60). Moreover, in the in vivo rat heart injury model induced by isoproterenol, ghrelin reduces apoptosis through activation of AMP-activated kinase (AMPK) while decreasing caspase-12 (61). Moreover, Iglesias et al. (62) described for the first time that isolated human cardiomyocytes synthesized and secreted ghrelin, protecting them against the apoptosis inducer cytosine arabinoside.
Anti-apoptotic effect of ghrelin in mucosa cells
Ghrelin is a modulator of the processes of gastric mucosal repair, and also a regulator of mucosal nitrous oxide system responsible for nitric oxide (NO) production. In the primary cell culture of rats, gastric mucosal anti-apoptotic effect of ghrelin is associated with reduction of caspase-3 activity by nitrosylation and the increase in constitutive nitric oxide synthase (cNOS) activity by proto-oncogene tyrosine-protein kinase (Src)/Akt activation (63). Ercan et al. (64) confirm also the impact of ghrelin on rat gastric mucosa (64). They have shown that ghrelin decreases the number of apoptotic cells and caspase-3 activity. Furthermore, ghrelin also has an impact on intestine mucosa. Herein, research on rat models has shown that rats fed with an elemental diet cause hypotrophy. Ghrelin inhibits apoptosis in jejunum and ileum, but also increases proliferation in the ileum (65). Another experiment in rat intestine has shown that ghrelin controls intestinal function through the regulation of intestinal apoptosis. In the small intestinal mucosa of fasting rats, ghrelin has anti-apoptotic effect, recovery of the villus height and decreased expression of caspase-3. Additionally, ghrelin also stimulates proliferation of mucosa cells (66).
Anti-apoptotic effect of ghrelin in neuronal cells
A very important action of ghrelin is its protective role in the brain. Stroke occurs when brain is deprived of oxygen and glucose. In ischemic cell death, the apoptosis mechanism is utilised, thus leading to ischemic injury. Ghrelin helps by increasing the Bcl-2/Bax ratio, preventing cytochrome c release and inhibiting caspase-3 activation (67). Ghrelin also prevents rat cortical neurons from injury induced by ischemia/reperfusion using this same mechanism, and additionally by increasing expression of heat shock protein (HSP70) and inhibiting caspases-8 and -9 (68). Neuroprotection against ischemic neuronal injury has been shown also using primary culture of rat cortical neurons exposed to oxygen. Therein, ghrelin prevents apoptosis by increasing Bcl-2 while decreasing Bax, and similar to previous models, inhibits also cytochrome c release and caspase-3 activation (69). This suggests its role in therapeutic strategy of cortex injury and glucose deprivation. Furthermore, ghrelin inhibits tunicamycin- or thapsigargin-triggered endoplasmic reticulum stress-induced apoptotic cell death in primary rat cortical neurons, where it acts on PI3K/Akt that leads to inactivation of glycogen synthase kinase 3 beta (GSK-3β) and Bcl-2-associated death promoter (BAD) (70). By the same signalling pathways, a similar effect is observed in rat models of hippocampal neurons with pilocarpine-induced apoptosis. Therein, ghrelin increases Bcl-2/Bax but decreases caspase-3 through PI3K/Akt kinase activation (71). Ghrelin may also protect retinal neurons against glaucomatous injury in rat model by blocking episcleral veins and inhibiting cleavage products of caspase-3, as well as reducing the number of TUNEL-positive cells (72). Another effect of ghrelin is attenuating the breakdown of the blood-brain barrier and apoptosis 24 hours following traumatic brain injury in mice model. This is accomplished by inhibiting apoptosis and caspases-3 activity, as well as increasing sterol uptake control protein 2 (UPC-2) (73). The PC12 cell line derived from pheochromocytoma of rat adrenal medulla is a suitable model for studying neuronal cell death on diabetes encephalopathy. In this model, high glucose-induced apoptosis is significantly inhibited by ghrelin treatment that leads to a decreased Toll-like receptor 4 (TLR4)/NF-κB pathway (74). In diabetes-induced pituitary cell death in rats, ghrelin reduces cell death, inducible nitric oxide synthase (iNOS) and active caspase-8, but increases prolactin, Bcl-2 and HSP70 contents in the pituitary (75). In hybrid MES23.5 cell line, 1-methyl-4-phenylpyridinium (MPP) - the same kind of drug used in mice to induce Parkinson’s disease causes apoptosis by increasing caspase-3 activity; ghrelin reverses this effect (76). Lastly, ghrelin also mediates apoptosis through the action of the apoptosis signal-regulating kinase 1 (ASK1) by a mechanism involving on induction of HSP70 expression (77).
Anti-apoptotic effect of ghrelin in cancer cells
There is also evidence that ghrelin has anti-apoptotic effect in cancer cells, which can have a negative effect on chemotherapy treatment. In colonic cancer HT-29 cells, ghrelin inhibits apoptosis caused by 5-fluorouracil by decreasing caspase-3 activity and increasing Bcl-2/Bax ratio (78). In canine mammary gland cancer, higher expression of ghrelin has been detected in cancer cell lines isolated from lung metastases (CMT-W1M and CMT-W2M) compared to those from primary tumours (CMT-W1 and CMT-W2). In this kind of tumour, ghrelin promotes proliferation and inhibits apoptosis (79). However, in PC3 prostate cancer cell line, ghrelin has a proliferative effect on the cells, but does not protect them from apoptosis (80). Anti-apoptotic effect of ghrelin in cancer sometimes has a positive effect. Tumours have an impact on muscle regeneration, where cancer cachexia is a syndrome associated with myofibre damage by apoptosis. In mouse C2C12 myoblasts and CT26 colon carcinoma cell models, ghrelin has been shown to prevent increased expression of TNF-α, c-Jun N-terminal kinases (JNK) and Bax/Bcl-2 ratio, as well as decreased phosphorylation of the Akt kinase, suggesting ghrelin’s role in treating cancer cachexia (81).
Anti-apoptotic effect of ghrelin in endothelial cells
Endothelial dysfunction caused by cell apoptosis is one of the most important diabetic vascular complications. Advanced glycation end products (AGEs) induce apoptosis of endothelial cells. In HUVEC, ghrelin inhibits apoptosis caused by AGEs and decreases caspase-3 activity by activation of ERK1/2 and Akt kinases (82). Li et al. (83) have shown on this same model that ghrelin utilises another signalling pathway, the cyclic guanosine monophosphate (cGMP)/NO. In case of high glucose-induced apoptosis in HUVEC, ghrelin can inhibit apoptosis by activation of kinases mTOR/P70S6K and increased ratio of Bcl-2/Bax (84). These results are also observed in HUVEC ECV-304 cell line where ghrelin decreased the caspase-3 level by activation of Akt kinase (85). Moreover, in human microvascular endothelial cells exposed to high glucose and lipid, ghrelin also decreases the number of apoptotic cells and inhibits caspase-3 activity; the authors propose that this action is possible by blocking ERK1/2, JNK1/2 and p38 signalling (86). All these results mean that ghrelin has a potential in preventing diabetic complications. Moreover, ghrelin also protects other endothelial cells, such as murine retinal microvascular endothelial cells (RMECs), from oxidative stress-induced apoptosis by sirtuin 1 (SIRT1) signalling pathway (87). In palmitate-induced apoptosis of rat aortic endothelial cells and hypoxia-induced injury of human pulmonary artery endothelial cells (HPAECs), apoptosis is inhibited by ghrelin, which acts by Akt kinase, increased expression of Bcl-2 and decreased expression of Bax (88, 89).
Anti-apoptotic effect of ghrelin in other cells
Ghrelin and its receptors are expressed in the adrenal gland, where they regulate human adrenal cortex function by increasing the proliferative activity but decreasing apoptotic deletion rate of zona glomerulosa (90). Ghrelin also has an impact on pancreatic beta-cells. Therein, prolonged exposure of pancreatic beta-cells to elevated levels of fatty acid is associated with apoptosis. In MIN6 pancreatic beta-cells line, ghrelin inhibits palmitate-induced apoptosis by activation of protein kinase B (PKB) and downregulation of JNK and Bax (91). In type I diabetes, major causes of cell destruction are apoptosis induced by serum starvation or interferon (IFN)-γ/TNF-α. In HIT-T15 beta-cells line, ghrelin inhibits apoptosis by cAMP upregulation and activation of Akt and ERK1/2 kinases (92). Similarly, Zhang et al. have shown that ghrelin increases Bcl-2 and inhibits Bax and caspase-3 activity (93). Through Akt kinases and with increasing caspase-3 level, ghrelin can also prevent lipopolysaccharide (LPS)-induced apoptosis in rat salivary gland acinar cells. In these cells, ghrelin increases cNOS activation by iNOS (63). Another major role of ghrelin is observed in pulmonary immune and epithelial cells, where ghrelin reduces lung injury and improves survival in sepsis. In LPS-induced apoptosis of human alveolar epithelial A549 cells, ghrelin acts by using known mechanisms that increase the ratio of Bax/Bcl-2 but decrease the expression of cleaved caspase-3 through phosphorylation of PI3K/Akt and ERK (94). In the same cell line, research has been conducted regarding pathological apoptosis in acute respiratory distress syndrome, in which iNOS activity in the lungs, NO production and level of protein S-nitrosylation are increased. However, ghrelin can decrease the intracellular NO production, protein S-nitrosylation and Bax protein expression, while increase Bcl-2 protein expression (95). Moreover, in bronchial epithelial 16HBE cells, ghrelin is shown to reverse the negative impact of high glucose level on cell survival. These results suggest the possible role of ghrelin in treating diabetic lung diseases (96). Bcl-2/Bax and caspase-3 regulation and activation of ERK kinases pathway are also used as a preventative mechanism of ghrelin in dexamethasone-induced rat insulinoma (INS-1) cell apoptosis (97). It is a well-known fact that in obesity, pro-inflammatory and pro-apoptotic cytokines such as TNF-α are upregulated. In human visceral adipocytes, ghrelin can decrease TNF-α-induced apoptosis and autophagy (98). Ghrelin prevents the intrinsic apoptotic pathway induced by serum deprivation through the activation of ERK1/2 and PI3K/Akt pathways in murine 3T3-L1 adipocytes (99). Similar results are observed in human HepG2 hepatocytes, ghrelin exerts anti-apoptotic effect by decreasing the activity of initiator (caspase 8), effector (caspase 3) and inflammatory (caspase 1) caspases (100). Ghrelin also decreases a number of apoptotic cells in mice model of doxorubicin-treated skeletal muscles (101). Finally, in osteoblastic MC3T3-E1 cell line, ghrelin inhibits apoptosis by suppressing caspase-3 activation (102, 103).
Pro-apoptotic effect of ghrelin
The pro-apoptotic effect of ghrelin is observed in causes of cancer, such as in colon adenocarcinoma and human lung cancer cell line H345, where this hormone also negatively impact cell proliferation (104, 105). Using TUNEL and ELISA assays, this same effect is also observed in aldosteronoma cell and SW-13, a human adrenal cortex/gland epithelial cell, or NCI-H295R, an Ang-II-responsive steroid-producing adrenocortical cell (106). In endothelial cells from rat brain microvessels (NECs), ghrelin is shown to decrease thymidine kinase (TK) and MAPK p42/p44 activities, suggesting it also has anti-angiogenic action (107). Sirini et al. have studied in bovine that ghrelin does not affect oocyte nuclear maturation and cumulus expansion area, but participates in cumulus cell death and apoptosis, with more profound effect observed in higher doses of ghrelin (108). Recent study indicated that the expression of cleaved-caspases-8, -9, and -3 were increased in ghrelin stimulation group compared with the control group in MH7A cells, suggesting that ghrelin promoted apoptosis by activating endogenous mitochondrial pathway and exogenous death receptor pathway (109).
Lack of effects of ghrelin on apoptosis
Study of Belloni et al. (106) showed that ghrelin (10–8 M) did not affect the apoptotic deletion rate in HUVEC, human normal adrenocortical cells, in rat osteoblasts, thymocytes or zona glomerulosa cells cultured in vitro.
The effect of ghrelin on cell apoptosis is mostly anti-apoptotic but there are also controversial reports documented no effect or pro-apoptotic action, which might stem from several reasons: first, cell type and culture conditions. For example, inhibitory effect of ghrelin on doxorubicin- induced apoptotic death was observed in H9c2 cardiomyocytes and endothelial cells (51), while in brain microvascular endothelial, NECs cultured on plastic discs in the presence of fetal calf serum (FCS) ghrelin promoted apoptotic death (107). Controversial, ghrelin protects human umbilical vein endothelial cells, HUVEC against high glucose-induced (84) or advanced glycation end products (AGEs)-induced (82) or AngII - induced apoptosis (110), while in HUVEC cultured on plastic discs in the presence of FCS ghrelin have no effect on apoptosis (106). Second, differential expression of ghrelin/GHSR subtypes may be involved in different effects of ghrelin in the apoptotic deletion rate of various cell type. The mRNA and protein expression of GHSR-1a has been demonstrated in the human and rat adrenal cortex (8), but not yet in adrenocortical tumor cells. It is difficult to discuss ghrelin effect on apoptosis depending on doses because range of ghrelin concentration used in experiments is huge, from 10–17 M (102) in in vitro study to 250 mg/kg (66) in in vivo experiments. In human plasma, the total ghrelin concentration is around 100 – 150 fmol/ml (7). Similar, time of cell incubation with ghrelin is difficult to analysis, it’s from 30 min in in vitro experiments (86) to 5 weeks in in vivo study (64).
Concluding remarks
Taken together, ghrelin exhibits a regulatory action on key cellular functions such as proliferation and apoptosis, by activation of different processes and regulation of many kinases. Specifically, it mediates apoptosis through the Akt kinase pathway, where it increases Bcl-2 protein expression but decreases Bax protein and caspase-3 activity (Fig. 3). Moreover, it mostly impacts organism functioning in a positive way, with exceptions in some types of cancer cells where ghrelin displays anti-apoptotic behaviour. These study provide novel insight into the understanding of the mechanisms of ghrelin in various cells protection and repair, and provide promising guidance for the clinical future application of ghrelin. Ghrelin has been administered in humans in multitude of studies and has a favourable safety profile with no side effects (111-114). Additionally, last interesting data demonstrated that ghrelin restored the loss of CD4 T cells by increasing their proliferation and expression of cyclin D1 and B1, while decreased the expression of p57 in sepsis mice, suggesting that ghrelin in can be developed as a promising therapeutic agent in sepsis (115).
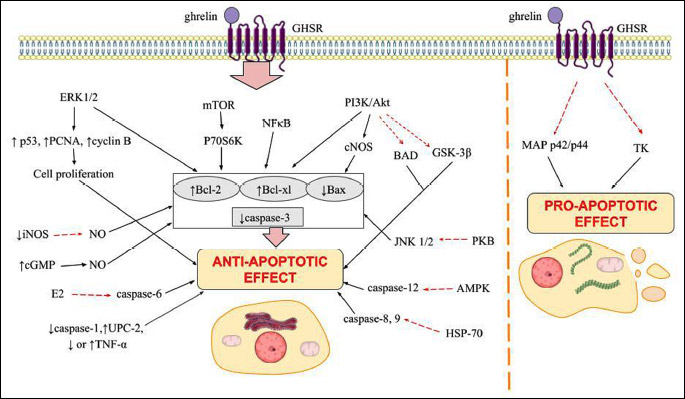
Abbreviations: ↑, increasing; ↓, decreasing; Akt, protein kinase B; AMPK, AMP activated kinase; BAD, Bcl-2-associated death promoter; Bcl-2, B-cell lymphoma 2; cGMP, cyclic guanosine monophosphate; cNOS, nitric oxide synthase; GHSR, growth hormone secretagogue receptor; GSK-3β, glycogen synthase kinase 3 beta; HSP70, heat shock protein; iNOS, inducible nitric oxide synthase; JNK, c-Jun N-terminal kinases; MEK-ERK, mitogen activated signaling pathway; mTOR, mammalian target of rapamycin kinase; NFκB, nuclear factor kappa B; NO, nitric oxide; P70S6K, ribosomal protein S6 kinase beta-1; PCNA, proliferating cell nuclear antigen; PI3K, phosphatidylinositide 3-kinases; PKB, protein kinase B; UPC-1, sterol uptake control protein.
Abbreviations: A549, adenocarcinomic human alveolar basal epithelial cells; AGEs, advanced glycation end products; AMPK, AMP activated kinase; Ang II, angiotensin II; ASK1, apoptosis signal-regulating kinase 1; BAD, Bcl-2-associated death promoter; Bcl-2, B-cell lymphoma 2; bcl-xs, B-cell lymphoma-extra small; bcl-xL, B-cell lymphoma-extra-large; Ca2+, calcium ions; cAMP, cyclic adenosino monophosphoran; cGMP, cyclic guanosine monophosphate; cNOS, nitric oxide synthase; E2, estradiol; GH, growth hormone; GHS-R, growth hormone secretagogue receptor; GSK-3β, glycogen synthase kinase 3 beta; HPAECs, human hypoxia-induced injury pulmonary artery endothelial cells; HSP70, heat shock protein; HUVEC, human umbilical vein endothelial cells; IL-2, interleukin 2; IL-3, interleukin 3; INF, interferon gamma; iNOS, inducible nitric oxide synthase; INS-1, rat insulinoma; JNK, c-Jun N-terminal kinases; LH, luteinizing hormone; LPS, lipopolysaccharide; MEK-ERK, mitogen activated signaling pathway; MH7A, rheumatoid arthritis fibroblast-like synoviocyte; MPP, methyl-4-phenylpyridinium; MPT, mitochondrial permeability transition; NECs, brain microvascular endothelial cells; NFκB, nuclear factor kappa B; NO, nitric oxide; OXT, oxytocin-neurophysin 1; PCNA, proliferating cell nuclear antigen; PGF, placenta growth factor; PI3K/Akt, phosphatidylinositide 3-kinases/protein kinase B; PKA, protein kinase A; PKB, protein kinase B; PTKs, protein tyrosine kinases; PLC, phospholipase C; SIRT1, sirtuin 1; Src, proto-oncogene tyrosine-protein kinase; RMECs, murine retinal microvascular endothelial cells; TK, thymidine kinase; TLR-4, Toll-like receptor 4; TNF, tumor necrosis factor; TNF-α/TNFR1, tumor necrosis factor receptor 1; TUNEL, terminal deoxynucleotidyl transferase (TdT) dUTP Nick-End Labeling; UPC-2, sterol uptake control protein 2;
Acknowledgements: This work was supported by the K/ZDS/008063, Jagiellonian University, Cracow, Poland.
Conflict of interests: None declared.
REFERENCES
- Jacobson MD, Weil M, Raff MC. Programmed cell death in animal development. Cell 1997; 88: 347-354.
- Kojima M, Hosoda H, Date Y, Nakazato M, Matsuo H, Kangawa K. Ghrelin is a growth hormone-releasing acylated peptide from stomach. Nature 1999; 402: 656-660.
- Smith RG, Van der Ploeg LH, Howard AD, et al. Peptidomimetic regulation of growth hormone secretion. Endocr Rev 1997; 18: 621-645.
- Castaneda TR, Tong J, Datta R, Culler M, Tschop MH. Ghrelin in the regulation of body weight and metabolism. Front Neuroendocrinol 2010; 31: 44-60.
- Patterson M, Murphy KG, leRoux CW, Ghatei MA, Bloom SR. Characterization of ghrelin-like immunoreactivity in human plasma. J Clin Endocrinol Metab 2005; 90: 2205-2211.
- Howard AD, Feighner SD, Cully DF, et al. A receptor in pituitary and hypothalamus that functions in growth hormone release. Science 1996; 273: 974-977.
- Tschop M, Weyer C, Tataranni PA, Devanarayan V, Ravussin E, Heiman ML. Circulating ghrelin levels are decreased in human obesity. Diabetes 2001; 50: 707-709.
- Gnanapavan S, Kola B, Bustin SA, Morris DG, McGee P, Fairclough P, et al. The tissue distribution of the mRNA of ghrelin and subtypes of its receptor, GHS-R, in humans. J Clin Endocrinol Metab 2002; 87: 2988. doi: 10.1210/jcem.87.6.8739
- Shiiya T, Nakazato M, Mizuta M, et al. Plasma ghrelin levels in lean and obese humans and the effect of glucose on ghrelin secretion. J Clin Endocrinol Metab 2002; 87: 240-244.
- Benso A, Calvi E, Gramaglia E, et al. Other than growth hormone neuroendocrine actions of ghrelin. Endocr Dev 2013; 25: 59-68.
- Hewson AK, Dickson SL. Systemic administration of ghrelin induces Fos and Egr-1 proteins in the hypothalamic arcuate nucleus of fasted and fed rats. J Neuroendocrinol 2000; 12: 1047-1049.
- Masuda Y, Tanaka T, Inomata N, et al. Ghrelin stimulates gastric acid secretion and motility in rats. Biochem Biophys Res Commun 2000; 276: 905-908.
- Perboni S, Inui A. Appetite and gastrointestinal motility. Role of ghrelin-family peptides. Clin Nutr 2010; 29: 227-234.
- Nagaya N, Kojima M, Uematsu M, et al. Hemodynamic and hormonal effects of human ghrelin in healthy volunteers. Am J Physiol Regul Integr Comp Physiol 2001; 280: R1483-R1487.
- Rak-Mardyla. Ghrelin role in hypothalamus-pituitary-ovarian axis. J Physiol Pharmacol 2013; 64: 695-704.
- Rak A, Gregoraszczuk EL. Modulatory effect of ghrelin in prepubertal porcine ovarian follicles. J Physiol Pharmacol 2008; 59: 781-793.
- Sirini MA, Anchordoquy JP, Quintana S, Furnus C, Relling AE, Anchordoquy JM. Expression of ghrelin and its receptor mRNA in bovine oocyte and cumulus cells. Int J Fertil Steril 2019; 12: 335-338.
- Dembinski A, Warzecha Z, Ceranowicz P, et al. Ghrelin attenuates the development of acute pancreatitisin rats. J Physiol Pharmacol 2003; 4: 561-573.
- Wang J, He L, Huwatibieke B, et al. Ghrelin stimulates endothelial cells angiogenesis through extracellular regulated protein kinases (ERK) signaling pathway. Int J Mol Sci 2018; 19: E 2530. doi: 10.3390/ijms19092530.
- Zhu J, Yao J, Huang R, Wang Y, Jia M, Huang Y. Ghrelin promotes human non-small cell lung cancer A549 cell proliferation through PI3K/Akt/mTOR/P70S6K and ERK signaling pathways. Biochem Biophys Res Commun 2018; 498: 616-620.
- Ye N, Wang L, Dou Z, Huang J. Ghrelin accelerates the cartilagic differentiation of rabbit mesenchymal stem cells through the ERK1/2 pathway. Cytotechnology 2018; 70: 415-421.
- Norbury CJ, Hickson ID. Cellular responses to DNA damage. Annu Rev Pharmacol Toxicol 2001; 41: 367-401.
- Kerr JF, Wyllie AH, Currie AR. Apoptosis: a basic biological phenomenon with wide-ranging implications in tissue kinetics. Br J Cancer 1972; 26: 239-257.
- Savill J, Fadok V. Corpse clearance defines the meaning of cell death. Nature 2000; 407: 784-788.
- Kurosaka K, Takahashi M, Watanabe N, Kobayashi Y. Silent cleanup of very early apoptotic cells by macrophages. J Immunol 2003; 171: 4672-4679.
- Igney FH, Krammer PH. Death and anti-death: tumour resistance to apoptosis. Nat Rev Cancer 2002; 2: 277-288.
- Green DR. Apoptotic pathways: ten minutes to dead. Cell 2005; 121: 671-674.
- Galluzzi L, Lopez-Soto A, Kumar S, Kroemer G. Caspases connect cell-death signaling to organismal homeostasis. Immunity 2016; 44: 221-231.
- Locksley RM, Killeen N, Lenardo MJ. The TNF and TNF receptor superfamilies: integrating mammalian biology. Cell 2001; 104: 487-501.
- Ashkenazi A, Dixit VM. Death receptors: signaling and modulation. Science 1998; 281: 1305-1308.
- Chicheportiche Y, Bourdon PR, Xu H, et al. TWEAK, a new secreted ligand in the tumor necrosis factor family that weakly induces apoptosis. J Biol Chem 1997; 272: 32401-32410.
- Peter ME, Krammer PH. Mechanisms of CD95 (APO-1/Fas)- mediated apoptosis. Curr Opin Immunol 1998; 10: 545-551.
- Suliman A, Lam A, Datta R, Srivastava RK. Intracellular mechanisms of TRAIL: apoptosis through mitochondrial-dependent and -independent pathways. Oncogene 2001; 20: 2122-2133.
- Rubio-Moscardo F, Blesa D, Mestre C, Siebert R, Balasas T, Benito A. Characterization of 8p21.3 chromosomal deletions in B-cell lymphoma: TRAIL-R1 and TRAIL-R2 as candidate dosage-dependent tumor suppressor genes. Blood 2005; 106: 3214-3222.
- Schulze-Osthoff K, Ferrari D, Los M, Wesselborg S, Peter ME. Apoptosis signaling by death receptors. Eur J Biochem 1998; 254: 439-459.
- Saelens X, Festjens N, Vande Walle L, van Gurp M, van Loo G, Vandenabeele P. Toxic proteins released from mitochondria in cell death. Oncogene 2004; 23: 2861-2874.
- Oltvai ZN, Korsmeyer SJ. Checkpoints of dueling dimers foil death wishes. Cell 1994; 79: 189-192.
- Jiang S, Chow SC, Nicotera P, Orrenius S. Intracellular Ca2+ signals activate apoptosis in thymocytes: studies using the Ca2+ ATPase inhibitor thapsigargin. Exp Cell Res 1994; 212: 84-92.
- de Vente JE, Kukoly CA, Bryant WO, et al. Phorbol esters induce death in MCF-7 breast cancer cells with altered expression of protein kinase C isoforms. Role for p53-independent induction of gadd-45 in initiating death. J Clin Invest 1995; 96: 1874-1886.e
- McConkey DJ, Orrenius S, Jondal M. Agents that elevate cAMP stimulate DNA fragmentation in thymocytes. J Immunol 1990; 145: 1227-1230.
- De A, Boyadjieva NI, Pastorcic M, Reddy BV, Sarkar DK. Cyclic AMP and ethanol interact to control apoptosis and differentiation in hypothalami beta-endorphin neurons. J Biol Chem 1994; 269: 26697-26705.
- Laneuville P, Timm M, Hudson AT. Bcr/abl expression in 32D cl3(G) cells inhibits apoptosis induced by protein tyrosine kinase inhibitors. Cancer Res 1994; 54: 1360-1366.
- Otani H, Erdos M, Leonard WJ. Tyrosine kinase(s) regulate apoptosis and bcl-2 expression in a growth factor-dependent cell line. J Biol Chem 1993; 268: 22733-22736.
- Zhang Y, Tounekti O, Akerman B, Goodyer CG, LeBlanc A. 17-beta-estradiol induces an inhibitor of active caspases. J Neurosci 2001; 21: RC176.
- Benco A, Sirotkin AV, Vasicek D, et al. Involvement of the transcription factor STAT1 in the regulation of porcine ovarian granulosa cell functions treated and not treated with ghrelin. Reproduction 2009; 138: 553-560.
- Gupta M, Dangi SS, Singh G, Sarkar M. Expression and localization of ghrelin and its receptor in ovarian follicles during different stages of development and the modulatory effect of ghrelin on granulosa cells function in buffalo. Gen Comp Endocrinol 2015; 210: 87-95.
- Sirotkin AV, Grossmann R, Maria-Peon MT, Roa J, Tena-Sempere M, Klein S. Novel expression and functional role of ghrelin in chicken ovary. Mol Cell Endocrinol 2006; 257-258: 15-25.
- Sirotkin AV, Grossmann R. Effects of ghrelin and its analogues on chicken ovarian granulosa cells. Domest Anim Endocrinol 2008; 34: 125-134.
- Rak-Mardyla A, Gregoraszczuk EL. ERK 1/2 and PI-3 kinase pathways as a potential mechanism of ghrelin action on cell proliferation and apoptosis in the porcine ovarian follicular cells. J Physiol Pharmacol 2010; 61: 451-458.
- Mousseaux D, Le Gallic L, Ryan J, et al. Regulation of ERK1/2 activity by ghrelin-activated growth hormone secretagogue receptor 1A involves a PLC/PKC epsilon pathway. Br J Pharmacol 2006; 148: 350-365.
- Baldanzi G, Filigheddu N, Cutrupi S, et al. Ghrelin and des-acyl ghrelin inhibit cell death in cardiomyocytes and endothelial cells through ERK1/2 and PI 3-kinase/AKT. J Cell Biol 2002; 159: 1029-1037.
- Liu Y, Liu Y, Li G, Chen Z, Gu G. Ghrelin protects the myocardium with hypoxia/reoxygenation treatment through upregulating the expression of growth hormone, growth hormone secretagogue receptor and insulin-like growth factor-1, and promoting the phosphorylation of protein kinase B. Int J Mol Med 2018; 42: 3037-3046.
- Kui L, Weiwei Z, Ling L, et al. Ghrelin inhibits apoptosis induced by high glucose and sodium palmitate in adult rat cardiomyocytes through the PI3K-Akt signaling pathway. Regul Pept 2009; 155: 62-69.
- Wang L, Lu Y, Liu X, Wang X. Ghrelin protected neonatal rat cardiomyocyte against hypoxia/reoxygenation injury by inhibiting apoptosis through Akt-mTOR signal. Mol Biol Rep 2017; 44: 219-226.
- Xu Z, Lin S, Wu W, et al. Ghrelin prevents doxorubicin-induced cardiotoxicity through TNF-alpha/NF-kappaB pathways and mitochondrial protective mechanisms. Toxicology 2008; 247: 133-138.
- Pei XM, Yung BY, Yip SP, Ying M, Benzie IF, Siu P. Desacyl ghrelin prevents doxorubicin-induced myocardial fibrosis and apoptosis via the GHSR-independent pathway. Am J Physiol Endocrinol Metab 2014; 306: E311-E323.
- Eid RA, Zaki MSA, Al-Shraim M, et al. Subacute ghrelin administration inhibits apoptosis and improves ultrastructural abnormalities in remote myocardium post-myocardial infarction. Peptides 2013; 48: 156-165.
- Yang C, Wang Y, Liu H, et al. Ghrelin protects H9c2 cardiomyocytes from angiotensin II-induced apoptosis through the endoplasmic reticulum stress pathway. J Cardiovasc Pharmacol 2012; 59: 465-471.
- Wang X, Yang C, Liu X, Yang P. Ghrelin alleviates angiotensin II-induced H9c2 apoptosis: impact of the miR-208 family. Med Sci Monit 2018; 24: 6707-6716.
- Yang C, Liu Z, Liu K, Yang P. Mechanisms of ghrelin anti-heart failure: inhibition of 7Ang II-induced cardiomyocyte apoptosis by down-regulating AT1R expression. PLoS One 2014; 9: e85785. doi: 10.1371/journal.pone.0085785
- Zhang GG, Cai HQ, Li YH, et al. Ghrelin protects heart against ERS-induced injury and apoptosis by activating AMP-activated protein kinase. Peptides 2013; 48: 156-165.
- Iglesias MJ, Pineiro R, Blanco M, et al. Growth hormone releasing peptide (ghrelin) is synthesized and secreted by cardiomyocytes. Cardiovasc Res 2004; 62: 481-488.
- Slomiany BL, Slomiany A. Constitutive nitric oxide synthase-mediated caspase-3 S-nitrosylation in ghrelin protection against Porphyromonas gingivalis-induced salivary gland acinar cell apoptosis. Inflammopharmacology 2010; 18: 119-125.
- Ercan S, Basaranlar G, Gungor NE, et al. Ghrelin inhibits sodium metabisulfite induced oxidative stress and apoptosis in rat gastric mucosa. Food Chem Toxicol 2013; 56: 154-161.
- de Segura IA, Vallejo-Cremades MT, Lomas J, et al. Exogenous ghrelin regulates proliferation and apoptosis in the hypotrophic gut mucosa of the rat. Exp Biol Med (Maywood) 2010; 235: 463-469.
- Park JM, Kakimoto T, Kuroki T, et al. Suppression of intestinal mucosal apoptosis by ghrelin in fasting rats. Exp Biol Med (Maywood) 2008; 233: 48-56.
- Hwang S, Moon M, Kim S, Hwang L, Ahn KJ, Park S. Neuroprotective effect of ghrelin is associated with decreased expression of prostate apoptosis response-4. Endocr J 2009; 56: 609-617.
- Miao Y, Xia Q, Hou Z, Zheng Y, Pan H, Zhu S. Ghrelin protects cortical neuron against focal ischemia/reperfusion in rats. Biochem Biophys Res Commun 2007; 359: 795-800.
- Chung H, Seo S, Moon M, Park S. Phosphatidylinositol-3-kinase/Akt/glycogen synthase kinase-3 beta and ERK1/2 pathways mediate protective effects of acylated and unacylated ghrelin against oxygen-glucose deprivation-induced apoptosis in primary rat cortical neuronal cells. J Endocrinol 2008; 198: 511-521.
- Chung H, Chung HY, Bae CW, Kim CJ, Park S. Ghrelin suppresses tunicamycin- or thapsigargin-triggered endoplasmic reticulum stress-mediated apoptosis in primary cultured rat cortical neuronal cells. Endocr J 2011; 58: 409-420.
- Zhang R, Yang G, Wang Q, Guo F, Wang H. Acylated ghrelin protects hippocampal neurons in pilocarpine-induced seizures of immature rats by inhibiting cell apoptosis. Mol Biol Rep 2013; 40: 51-58.
- Zhu K, Zhang ML, Liu ST, et al. Ghrelin attenuates retinal neuronal autophagy and apoptosis in an experimental rat glaucoma model. Invest Ophthalmol Vis Sci 2017; 58: 6113-6122.
- Lopez NE, Gaston L, Lopez KR, et al. Early ghrelin treatment attenuates disruption of the blood brain barrier and apoptosis after traumatic brain injury through a UCP-2 mechanism. Brain Res 2012; 1489: 140-148.
- Liu X, Xiao Q, Zhao K, Gao Y. Ghrelin inhibits high glucose-induced PC12 cell apoptosis by regulating TLR4/NF-κB pathway. Inflammation 2013; 36: 1286-1294.
- Granado M, Chowen JA, Garcia-Caceres C, et al. Ghrelin treatment protects lactotrophs from apoptosis in the pituitary of diabetic rats. Mol Cell Endocrinol 2009; 309: 67-75.
- Dong J, Song N, Xie J, Jiang H. Ghrelin antagonized 1-methyl-4-phenylpyridinium (MPP(+))-induced apoptosis in MES23.5 cells. J Mol Neurosci 2009; 37: 182-189.
- Yang M, Hu S, Wu B, Miao Y, Pan H, Zhu S. Ghrelin inhibits apoptosis signal-regulating kinase 1 activity via upregulating heat-shock protein. Biochem Biophys Res Commun 2007; 359: 373-378.
- He XT, Fan XM, Zha XL. Ghrelin inhibits 5-fluorouracil-induced apoptosis in colonic cancer cells. J Gastroenterol Hepatol 2011; 26: 1169-1173.
- Majchrzak K, Pawłowski KM, Orzechowska EJ, et al. A role of ghrelin in canine mammary carcinoma cells proliferation, apoptosis and migration. BMC Vet Res 2012; 8: 170. doi: 10.1186/1746-6148-8-170
- Yeh AH, Jeffery PL, Duncan RP, Herington AC, Chopin LK. Ghrelin and a novel preproghrelin isoform are highly expressed in prostate cancer and ghrelin activates mitogen-activated protein kinase in prostate cancer. Clin Cancer Res 2005; 11: 8295-8303.
- Zeng X, Chen S, Lin Y, Ke Z. Acylated and unacylated ghrelin inhibit apoptosis in myoblasts cocultured with colon carcinoma cells. Oncol Rep 2018; 39: 1387-1395.
- Xiang Y, Li Q, Li M, Wang W, Cui C, Zhang J. Ghrelin inhibits AGEs-induced apoptosis in human endothelial cells involving ERK1/2 and PI3K/Akt pathways. Cell Biochem Funct 2011; 29: 149-155.
- Li P, Liu Y, Xiang Y, Lin M, Gao J. Ghrelin protects human umbilical vein endothelial cells against advanced glycation end products-induced apoptosis via NO/cGMP signaling. Int J Clin Exp Med 2015; 8: 15269-15275.
- Zhu J, Zheng C, Chen J, et al. Ghrelin protects human umbilical vein endothelial cells against high glucose-induced apoptosis via mTOR/P70S6K signaling pathway. Peptides 2014; 52: 23-28.
- Zhao H, Liu G, Wang Q, et al. Effect of ghrelin on human endothelial cells apoptosis induced by high glucose. Biochem Biophys Res Commun 2007; 362: 677-681.
- Liao P, Yang D, Liu D, Zheng Y. GLP-1 and ghrelin attenuate high glucose/high lipid-induced apoptosis and senescence of human microvascular endothelial cells. Cell Physiol Biochem 2017; 44: 1842-1855.
- Shimada T, Furuta H, Doi A, et al. Des-acyl ghrelin protects microvascular endothelial cells from oxidative stress-induced apoptosis through sirtuin 1 signaling pathway. Metabolism 2014; 63: 469-474.
- Zhang D, Wang W, Zhou D, et al. Ghrelin inhibits apoptosis induced by palmitate in rat aortic endothelial cells. Med Sci Monit 2010; 16: BR396-BR403.
- Yang D, Liu Z, Zhang H, Luo Q. Ghrelin protects human pulmonary artery endothelial cells against hypoxia-induced injury via PI3-kinase/Akt. Peptides 2013; 42: 112-117.
- Mazzocchi G, Neri G, Rucinski M, et al. Ghrelin enhances the growth of cultured human adrenal zona glomerulosa cells by exerting MAPK-mediated proliferogenic and antiapoptotic effects. Peptides 2004; 25: 1269-1277.
- Wang W, Zhang D, Zhao H, et al. Ghrelin inhibits cell apoptosis induced by lipotoxicity in pancreatic beta-cell line. Regul Pept 2010; 161: 43-50.
- Granata R, Settanni F, Biancone L, et al. Acylated and unacylated ghrelin promote proliferation and inhibit apoptosis of pancreatic beta-cells and human islets: involvement of 3',5'-cyclic adenosine monophosphate/protein kinase A, extracellular signal-regulated kinase 1/2, and phosphatidyl inositol 3-Kinase/Akt signaling. Endocrinology 2007; 148: 512-529.
- Zhang Y, Ying B, Shi L, et al. Ghrelin inhibit cell apoptosis in pancreatic beta cell line HIT-T15 via mitogen-activated protein kinase/phosphoinositide 3-kinase pathways. Toxicology 2007; 237: 194-202.
- Huang C, Zheng H, He W, et al. Ghrelin ameliorates the human alveolar epithelial A549 cell apoptosis induced by lipopolysaccharide. Biochem Biophys Res Commun 2016; 474: 83-90.
- Zeng M, Huang C, Zheng H, Chen Q, He W, Deng Y. Effects of ghrelin on iNOS-derived NO promoted LPS-induced pulmonary alveolar epithelial A549 cells apoptosis. Cell Physiol Biochem 2018; 49: 1840-1855.
- Liu X, Chen D, Wu Z, et al. Ghrelin inhibits high glucose-induced 16HBE cells apoptosis by regulating Wnt/β-catenin pathway. Biochem Biophys Res Commun 2016; 477: 902-907.
- Zhang C, Li L, Zhao B, et al. Ghrelin protects against dexamethasone-induced INS-1 cell apoptosis via ERK and p38MAPK signaling. Int J Endocrinol 2016; 2016: 4513051. doi: 10.1155/2016/4513051
- Rodriguez A, Gomez-Ambrosi J, Catalan V, et al. The ghrelin O-acyltransferase-ghrelin system reduces TNF-α-induced apoptosis and autophagy in human visceral adipocytes. Diabetologia 2012; 55: 3038-3050.
- Kim MS, Yoon CY, Jang PG, et al. The mitogenic and antiapoptotic actions of ghrelin in 3T3-L1 adipocytes. Mol Endocrinol 2004; 18: 2291-301.
- Ezquerro S, Mocha F, Fruhbeck G, et al. Ghrelin reduces TNF-α-induced human hepatocyte apoptosis, autophagy and pyroptosis: role in obesity-associated NAFLD. J Clin Endocrinol Metab 2019; 104: 21-37.
- Yu AP, Pei XM, Sin TK, et al. Acylated and unacylated ghrelin inhibit doxorubicin-induced apoptosis in skeletal muscle. Acta Physiol (Oxf) 2014; 211: 201-213.
- Kim SW, Her SJ, Park SJ, et al. Ghrelin stimulates proliferation and differentiation and inhibits apoptosis in osteoblastic MC3T3-E1 cells. Bone 2005; 37: 359-369.
- Hao Y, Liu C, Huang J, et al. Ghrelin protects against depleted uranium-induced apoptosis of MC3T3-E1 cells through oxidative stress-mediated p38-mitogen-activated protein kinase pathway. Toxicol Appl Pharmacol 2016; 290: 116-225.
- Bonfili L, Cuccioloni M, Cecarini V, et al. Ghrelin induces apoptosis in colon adenocarcinoma cells via proteasome inhibition and autophagy induction. Apoptosis 2013; 18: 1188-1200.
- Cassoni P, Allia E, Marrocco T, et al. Ghrelin and cortistatin in lung cancer: expression of peptides and related receptors in human primary tumors and in vitro effect on the H345 small cell carcinoma cell line. J Endocrinol Invest 2006; 29: 781-790.
- Belloni AS, Macchi C, Rebuffat P, et al. Effect of ghrelin on the apoptotic deletion rate of different types of cells cultured in vitro. Int J Mol Med 2004; 14: 165-167.
- Baiguera S, Conconi MT, Guidolin D, et al. Ghrelin inhibits in vitro angiogenic activity of rat brain microvascular endothelial cells. Int J Mol Med 2004; 14: 849-854.
- Sirini MA, Anchordoquy JM, Anchordoquy JP, et al. The presence of acylated ghrelin during in vitro maturation of bovine oocytes induces cumulus cell DNA damage and apoptosis, and impairs early embryo development. Zygote 2017; 25: 601-611.
- Ma J, Wang X, Lv T, et al. Effects of ghrelin on the apoptosis of rheumatoid arthritis fibroblast-like synoviocyte MH7A cells. Biol Pharm Bull 2019; 4: 158-163.
- Deng B, Fang L, Chen X, Chen M, Xie X. Effect of ghrelin on angiotensin II induced human umbilicus vein endothelial cell oxidative stress and endothelial cell injury. Zhong Nan Da Xue Xue Bao Yi Xue Ban 2010; 35: 1037-1047.
- Narula T, deBoisblanc BP. Ghrelin in critical illness. Am J Respir Cell Mol Biol 2015; 53(4): 437-442.
- Garin MC, Burns CM, Kaul S, Cappola AR. The human experience with ghrelin administration. Clinical review. J Clin Endocrinol Metab 2013; 98: 1826-1837.
- Slupecka-Ziemilska M, Wolinski J, Herman AP, Romanowicz K, Dziegielewska Z, Borszewska-Kornacka MK. Influence of preterm delivery on ghrelin and obestatin concentrations in maternal plasm, milk and their expression in mammary epithelial cells. J Physiol Pharmacol 2017; 68: 693-698.
- Zatorski H, Mosinska P, Storr M, Fichna J. Relamorelin and other ghrelin receptor agonists - future options for gastroparesis, functional dyspepsia and proton pump inhibitors-resistant non-erosive reflux disease. J Physiol Pharmacol 2017; 68: 797-805.
- Zhou M, Aziz M, Ochani M, Yang WL, Sharma A, Wang P. The protective role of human ghrelin in sepsis: Restoration of CD4 T cell proliferation. PLoS One 2018; 13: e0201139. doi: 10.1371/journal.pone.0201139
A c c e p t e d : February 28, 2019