Doxorubicin (DOX) and docetaxel (DTX) are widely used in chemotherapy of different types of cancers. Combination therapy employing these drugs has been proven particularly effective in the treatment of breast cancer (1). Anthracycline doxorubicin is a topoisomerase II inhibitor, which intercalates into DNA (2, 3). Its toxic effects can also be mediated through other mechanisms including induction of apoptosis and free radical formation (4-6).
The principal activity of docetaxel is the inhibition of microtubule depolymerization that causes aberrant mitosis and often leads to cell death (7). Additionally, docetaxel may evoke oxidative stress (8).
Combination of DOX and DTX is clinically effective against many cancers, however, potentiation of side-effects (
e.g. cardiotoxicity) is observed in most of the patients (9-11). This undesirable effect, along with others, such as neurotoxicity, hepatotoxicity or nephrotoxicity is mainly related to free radical formation and oxidative damage to biological macromolecules (12). Therefore, the inclusion of compounds with antioxidant activity to the standard DOX-DTX chemotherapy could be a promising strategy for reduction of oxidative stress and toxicity in non-targeted tissues.
Nitroxides, which are nontoxic, cell-permeable, low molecular weight and stable free radicals, have been proposed as a new class of universal antioxidants (13). They possess the ability to undergo one-electron reduction/oxidation and shuttle between three oxidation states forming corresponding hydroxylamines/oxoammonium cations (14, 15). It has been shown that nitroxides can act catalytically as SOD mimics and pro-catalysts by stimulating catalase like activities in heme proteins (16, 17). Moreover, nitroxides, due to their free radical nature can interrupt chain propagating lipid peroxidation reactions, thus prevent lipid damage (18).
Promising results from the
in vitro,
ex vivo and
in vivo
studies, conducted in our laboratory (19-21) prompted us to further investigation
of antioxidant properties of nitroxides as modulators of oxidative stress, generated
in vivo by anticancer drugs. Excess of reactive oxygen species (ROS)
in non-targeted tissues has been proven to be a key factor in frequent side
effects developed by patients undergoing chemotherapy (22).
In this study, we evaluated the ability of pyrroline nitroxyl derivative pirolin
(3-carbamoyl-2,2,5,5-tetramethylpyrroline-1-oxyl) (PL) (
Fig. 1) to reduce
oxidative stress generated in blood plasma of rats bearing experimental mammary
tumor and treated with a combination of doxorubicin and docetaxel, each used
at two concentrations. For this purpose, we have measured the main biomarkers
of oxidative stress such as lipid peroxidation products (hydroperoxides, TBARS),
thiol and carbonyl groups, activity of superoxide dismutase and non-enzymatic
antioxidant capacity, NEAC).
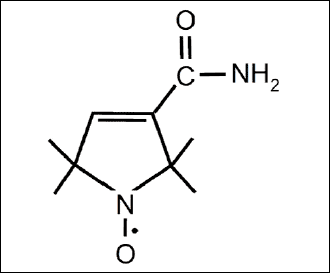 |
Fig. 1. Chemical structure
of pirolin. |
In order to test another hypothesis that pirolin can have both pro- and anti-oxidative effects depending on experimental conditions we additionally evaluated activity of this nitroxide administered as a single agent according to two different regimens - at 3 separate doses given at 3-week intervals (regimen A) or every day over a 2-week period (regimen B).
It is believed that generation of ROS as a consequence of DMBA metabolism plays a role in the process of carcinogenesis. Thus, we also tested differences in the oxidative stress-related parameters between healthy rats and rats with DMBA-induced tumors.
MATERIALS AND METHODS
Chemicals
Doxorubicin and docetaxel were purchased from Sequoia Research Products Ltd., Pangbourne, United Kingdom. Pirolin was synthesized from 3-carbamoyl-2,2,5,5-tetra-methylpyrroline according to the protocol designed by Rozantsev (23). Pirolin crystals were recrystallized from ethanol, and the melting point of the crystals was measured as 203-204°C. Butylated hydroxytoluene (BHT; 2,6-di-tert-butyl-4-hydroxytoluene), bovine serum albumin (BSA), Ellman`s reagent (di-thio-nitrobenzoic acid, DTNB), ferrous ammonium sulfate, 7,12-dimethylbenz(a)anthracene and D-sorbitol were purchased from Sigma-Aldrich. Adrenaline and xylenol orange were obtained from MP Biomedicals. Hydrogen peroxide and 2-thiobarbituric acid (TBA) were purchased from POCH, Gliwice, Poland. All other reagents were of the highest purity available. Deionized Q water was used in the preparation of all solutions (Millipore Corp., Bedford).
Animal study
1. Animals
The study was carried out on the female Sprague-Dawley rats of average weight
of 180 g (161-213 g). Free access to water and a standard feed for laboratory
rodents were provided
ad libitum for all animals. All experiments on
rats were performed according to the guiding rules published by the US National
Research Council (Guide for the Care and Use of Laboratory Animals, National
Academic Press, Washington, DC, ed. 7, 1996); the guidelines of the European
Community for the Use of Experimental Animals (L358-86/609/EEC) and the Guiding
Principles in the Use of Animals in Toxicology (1989). Experimental design was
approved by an appropriate institutional, local ethics committee.
2. Tumor induction
The induction of mammary tumors was conducted with 7,12-dimethylbenz(a)anthrance
(DMBA) as described by Barros
et al. (24). At the age of 50 days, rats
were given a single intragastric dose of 20 mg of DMBA diluted in 1 ml of olive
oil. From that moment on, the animals were examined on a weekly basis for the
appearance of palpable mammary tumors. During 10 weeks after carcinogen administration
the appearance, location and size of tumors were regularly recorded as described
by Whitsett
et al. (25). Rats that failed to develop tumor by 10 weeks
after the administration of the carcinogen were discarded. Animals, administered
with olive oil only, served as a negative control and were marked as healthy
controls. Rats that developed mammary tumors were divided into two groups marked
as: untreated controls - rats receiving a vehicle (5% glucose) and six experimental
groups (6 animals each) - rats receiving different treatment according to
Fig.
2. All investigated compounds were suspended in 5% glucose and administered
intraperitoneally.
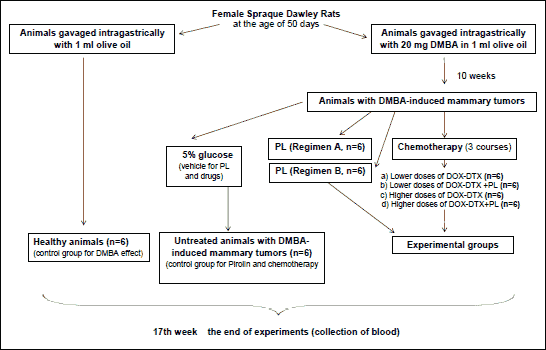 |
Fig. 2. Graphic description
of experiment. |
3. Treatment with pirolin as a single agent
Two regimens for pirolin treatment were tested:
Regimen A: 10 mg pirolin/kg b.w., 3 administrations at 3-week intervals;
Regimen B: 10 mg pirolin/kg b.w., daily, for 2 weeks.
4. Chemotherapy with DOX-DTX combination in conjunction with pirolin
a) chemotherapy with lower drug doses (2.5 mg DOX/kg b.w. followed by 3.75 mg DTX/kg b.w. one hour later, 3 administrations, at 3-week intervals);
b) chemotherapy with lower drug doses and inclusion of pirolin (2.5 mg DOX/kg b.w. and 10 mg PL/kg b.w. followed by 3.75 mg DTX/kg b.w. one hour later, 3 administrations, at 3-week intervals);
c) chemotherapy with higher drug doses (5 mg DOX/kg b.w. followed by 7.5 mg DTX/kg b.w. one hour later, 3 administrations, at 3-week intervals);
d) chemotherapy with higher drug doses and inclusion of pirolin (5 mg DOX/kg b.w. and 10 mg PL/kg b.w. followed by 7.5 mg DTX/kg b.w. one hour later, 3 administrations, at 3-week intervals).
Pirolin and drug doses were selected as the most optimal on the basis of the pilot experiments (19).
Electron paramagnetic resonance measurements
Electron paramagnetic resonance (EPR) technique was employed in order to check stability of pirolin in solution and its possible interactions with doxorubicin (during experiments PL and DOX were administered to animals together in one solution). EPR signal of the nitroxide was measured on ESP-300E spectrometer (Bruker, Germany) working in X-band and equipped with the OS-9 operating system. The following settings of the spectrometer were used: microwave frequency 9.73 GHz, microwave power 20 mW, central field 3480 G, field modulation amplitude 80 G, field modulation frequency 100 kHz. The signal of pirolin was measured for both its water solution and its 1:1 mixture with doxorubicin. The spectra were recorded at the time points: 0 h, 3 h and 24 h. Capillaries with compounds were kept in a water bath set to 20°C. Since the EPR signal of nitroxides is well correlated with their concentration, the amplitude of the middle field line was taken for calculations. We did not perform measurements for interaction of DTX with PL due to insolubility of taxanes in water solutions and inability of their molecules to directly participate in any redox reactions. It should be mentioned that both compounds docetaxel and pirolin were administered to animals in a separate solution at 1-hour interval.
Preparation of plasma samples
On the 4
th day after the last course of chemotherapy,
the rats were anaesthetized and killed off by cervical dislocation. Samples
of venous blood were collected in tubes containing EDTA. Plasma was separated
by centrifugation at 1500×g for 10 min. Each sample was analyzed at least in
triplicate.
Estimation of biomarkers of oxidative stress
1. Thiol groups
Total concentration of thiol groups (-SH) was measured spectrophotometrically
at 412 nm according to Ellman's method (26). An aliquot of 100 µl of blood plasma
was mixed with 100 µl of 10% SDS and 800 µl of 10 mM phosphate buffer (pH 8)
and the absorbance of the solution was measured at 412 nm (A0) against blank.
Then, 100 µl of DTNB was added followed by incubation at 37°C for 60 min. After
incubation the absorbance of the sample was measured again at 412 nm (A1). The
thiol group concentration was calculated from a A1-A0 subtraction using a molar
extinction coefficient of 13.6×10
3 M
-1×cm
-1.
Results were expressed as nanomoles of thiol groups per mg of protein.
2. Protein carbonyl groups
Protein carbonyl groups were measured by the method of Levine
et al.
(27) using 2,4-dinitrophenylhydrazine (DNPH). Appropriate volume of blood plasma
(containing 1 mg protein) was treated with 1 ml of a 10 mM 2,4-dinitro-phenylhydrazine
dissolved in 2.5 M HCl. Samples were then incubated for 1 h at room temperature,
in the dark and stirred every 15 min. After incubation, 1 ml of 20% trichloroacetic
acid was added to each sample, and they were left to precipitate on ice for
10 min. Following centrifugation (3000×g, 10 min, room temperature), the protein
pellet was washed three times with ethanol and ethyl acetate (1:1 ratio, v/v),
suspended in 1 ml of 6 M guanidine-HCl and incubated at 37°C for 30 min. The
carbonyl groups were determined from the absorbance at 370 nm (molar absorption
coefficient

=21×10
3
M
-1×cm
-1). Each
sample was read against the blank (6 M guanidine-HCl). Results were expressed
as nanomoles of carbonyl groups per mg of protein.
3. Superoxide dismutase activity
Superoxide dismutase (SOD) activity was assayed in terms of inhibition of adrenaline self-oxidation at 480 nm (28). The maximum rate of adrenaline oxidation (0.025 A/min) was taken as 0% inhibition. The volume of plasma which caused 50% inhibition of adrenaline autooxidation was regarded as containing one unit of SOD. The activity of SOD was calculated and expressed in international units per milligram of protein.
4. Plasma non-enzymatic antioxidant capacity
The non-enzymatic antioxidant capacity (NEAC) of blood plasma was performed by 1,1-diphenyl-2-picrylhydrazyl (DPPH) reduction assay (29). 20 µl of blood plasma was added to 400 µl of 0.1 mM methanol solution of DPPH. The samples were incubated for 30 min at room temperature and absorbance was measured at 517 nm. The antioxidant capacity of the plasma was expressed as nmol Trolox equivalents (TE) per mg of protein.
5. Hydroperoxides
Concentration of hydroperoxides was determined by FOX-1 method with the later
modification (30). FOX-1 reagent contained 125 µM xylenol orange and 100 mM
sorbitol in 25 mM H
2SO
4
and was freshly prepared each time before the use by the addition of ammonium
ferrous sulfate to the final concentration of 250 µM. To perform FOX-1 assay,
an aliquot of 100 µl of blood plasma was mixed with 1 ml of working FOX-1 reagent
and vortexed. The samples were centrifuged (20°C, 3000×g, 10 min.) After 30
min incubation in the dark (room temperature) absorbance of the supernatant
was measured spectrophotometrically at 560 nm against blank. The amount of hydroperoxides
was estimated from a standard curve for H
2O
2.
6. Thiobarbituric acid-reactive substances
Lipid peroxidation was assayed by determining the production of thiobarbituric
acid-reactive substances (TBARS) (31). Equal volumes of plasma, 15% (w/v) trichloroacetic
acid in 0.25 M HCl and 0.37% (w/v) 2-thiobarbituric acid in 0.25 M HCl containing
2% BHT were mixed. The reaction mixture was incubated at 100°C for 10 min. Finally,
the samples were allowed to cool, centrifuged (3000×g, 5 min, 20°C), and the
absorbance of supernatant was measured at 535 nm against blank. TBARS content
was calculated from

=1.56×10
5
M
-1×cm
-1 and expressed
as nanomoles of TBARS per milligram of protein.
7. Assessment of protein content
The amount of protein was assayed by Lowry method (32) from the standard curve for bovine serum albumin.
Statistical analysis
All values were expressed as a median and lower-upper quartile range. Normality
of data distribution was checked by Shapiro-Wilk's test. Homogeneity of variance
was evaluated using the Levene's test. The statistical significance between
two groups (control rats vs. rats with DMBA-induced tumors) was assessed by
the Mann-Whitney
U test. The statistical significance in experiment with
two different regimens of PL treatment was estimated using one-way ANOVA and
post hoc Tukey-Kramer test for multiple comparisons (more than two groups).
For data that showed non-homogeneity of variance, the non-parametric Kruskal-Wallis
median test and
post hoc all pairwise Connover-Inman test were used.
In section "Chemotherapy with DOX-DTX combination in conjunction with pirolin"
of the experimental study, the parametric two-way analysis of variance was used.
This test enables the testing of possible interactions between compounds. If
the interaction occurs, the two-way, nested analysis of variance is used, assuming
that the impact of drugs is at the higher level of importance than the impact
of pirolin. Differences were considered to be significant at least at p<0.05.
The Statistica software (StatSoft Inc., Tulsa, OK, USA) and StatsDirect software
(StatsDirect Ltd., England) were used for statistical evaluations.
RESULTS
Electron paramagnetic resonance measurements
After 24 h incubation at 20°C of Pirolin solution or its mixture with doxorubicin we did not detect any significant changes in the nitroxide EPR signal (data not shown). Therefore, we conclude that in conditions of our experiments pirolin was rather stable and no detectable direct interaction between the molecules of a nitroxide and doxorubicin occurred.
Changes in oxidative stress-related parameters in rats with 7,12-dimethylbenz(a)anthracene-induced tumors
In rats with DMBA-induced tumors we have found significant oxidative damage
to proteins and lipids manifested by a substantial increase in amounts of hydroperoxides
(2-fold), thiol groups (2.5-fold), TBARS (about 1.5-fold) and carbonyl groups
(1.1-fold). At the same time depletion of SOD activity and more than 4-fold
enhancement of non-enzymatic antioxidant activity were observed (
Table 1).
These results suggest development of oxidative stress in the plasma of rats
bearing DMBA-induced tumors due to the process of carcinogenesis.
Changes
in oxidative stress-related parameters in rats with 7,12-dimethylbenz(a)anthracene-induced
tumors treated with pirolin as a single agent
In order to evaluate the ability of pirolin to attenuate oxidative stress generated
in the plasma of rats during DMBA-induced carcinogenesis we administered a nitroxide
alone according to two different protocols. Administration of a nitroxide at
3-week intervals caused a considerable elevation (p<0.01) of all investigated
parameters (except of -SH groups) (
Table 1). This suggests a pro-oxidative
effect of pirolin under these experimental conditions. Results obtained for
protocol B (daily 2-week treatment) were less consistent and suggested both
its pro-oxidative and antioxidative activities depending on the considered parameter.
Nitroxide caused an increase in the - SH group content and a decrease in the
amount of carbonyl groups, which might suggests its protective role on plasma
proteins. On the other hand pirolin elevated TBARS about a 3-fold which rather
pointed to significant peroxidation of plasma lipids. Concurrently no visible
effect of pirolin on SOD activity and non-enzymatic antioxidant activity was
found (
Table 1).
Table 1. Changes
in parameters of oxidative stress in healthy rats and rats with DMBA-induced
mammary tumors (untreated and treated with a nitroxyl derivative pirolin).
All data are presented as a median and lower-upper quartile range. Healthy
rats - rats given a single intragastric dose of 1 ml of olive oil (a vehicle
for DMBA). Rats with DMBA-induced mammary tumors - rats that developed
mammary tumors after receiving a single intragastric dose of 20 mg of
DMBA diluted in 1 ml of olive oil. Untreated - rats with DMBA-induced
mammary tumors injected with a vehicle for pirolin (5% glucose), NS -
non-significant. |
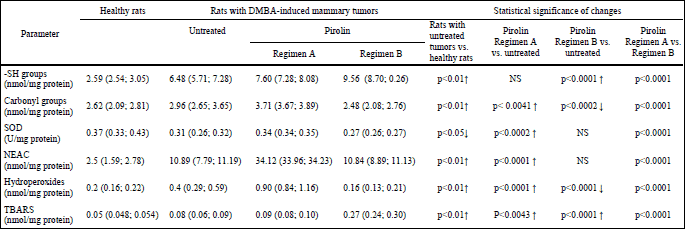 |
Taking into consideration inconsistency of results obtained for protocol B of pirolin treatment and our earlier data on the protective effect of other nitroxide derivative (TEMPO) on damage to rat cardiomyocytes caused by doxorubicin
in vivo, we decided to use protocol A in the further experiments. Earlier we had found that in spite of its pro-oxidative effect when applied as a single agent (an increase in TBARS), Tempo significantly attenuated lipid peroxidation induced by doxorubicin in cardiac muscle of rats when it was given in conjunction with DOX (33).
Changes in oxidative stress-related parameters in rats bearing 7,12-dimethylbenz(a)anthracene-induced tumors treated with a combination of anticancer drugs doxorubicin and docetaxel alone and in conjunction with pirolin
1. Changes in the -SH group content
Treatment with anticancer drugs caused a considerable decrease in the content
of -SH groups, which evidenced induction of oxidative stress and significant
thiol depletion during chemotherapy. This effect was independent on the drug
doses. Inclusion of pirolin to the treatment had no influence on these changes
(
Fig. 3, 3A).
 |
Fig. 3. Effect of administration
of combination of doxorubicin-docetaxel and combination of doxorubicin-docetaxel-pirolin
on thiols and oxidation of proteins in blood plasma of rats bearing DMBA-induced
mammary tumors: A: -SH groups, B: protein carbonyl groups.
Each data point is presented as a median and lower-upper quartile range.
*p<0.05 in relation to untreated animals. Untreated - rats with
DMBA-induced mammary tumors injected with a vehicle for the investigated
compounds (5% glucose); DOX-DTX (l) - rats treated with combination
of lower doses of DOX (2.5 mg/kg b.w.) and DTX (3.75 mg/kg b.w.); DOX-DTX
(l)+PL - rats treated with combination of lower doses of DOX (2.5
mg/kg b.w.) and DTX (3.75 mg/kg b.w.) with addition of pirolin (10 mg/kg
b.w.); DOX-DTX (h) - rats treated with combination of higher doses
of DOX (5 mg/kg b.w.) and DTX (7.5 mg/kg b.w.); DOX-DTX (h)+PL
- rats treated with combination of higher doses of DOX (5 mg/kg b.w.)
and DTX (7.5 mg/kg b.w.) with addition of pirolin (10 mg/kg b.w.). |
2. Estimation of protein carbonyl groups
The amount of protein carbonyl groups in blood plasma of healthy rats was estimated
as 2.62 (2.09; 2.81) nmol/mg protein (
Table 1). Similar amount of carbonyl
groups was found in rats receiving low doses of DOX-DTX independently whether
the drugs were used alone or in conjunction with pirolin. Instead, an elevated
content of carbonyl groups was found in rats receiving higher doses of DOX-DTX
and rats administered with higher doses of DOX-DTX with the addition of PL.
Pirolin displayed some kind of protective activity only in combination with
the higher drug doses as the amount of carbonyl groups evoked by higher doses
of DOX and DTX in respect to untreated controls was reduced by 25% when pirolin
was included in the treatment (
Fig. 3, 3B).
3. Superoxide dismutase and non-enzymatic antioxidant capacity activities
Elevated SOD activity in respect to untreated controls was found in rats receiving
chemotherapy (
Fig. 4, 4A). Addition of PL to the DOX-DTX treatment, employing
lower drug doses, caused further elevation of SOD activity while no such effect
was found for the combination of a nitroxide with the higher drug doses.
Significant elevation of NEAC, expressed in nanomole equivalents of Trolox,
was observed in all experimental groups as compared to untreated controls (
Fig.
4, 4B).
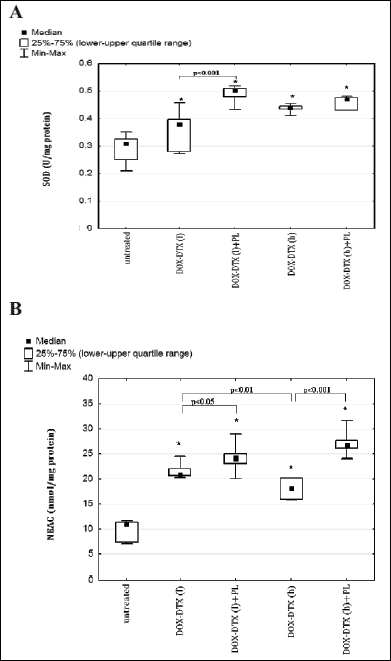 |
Fig. 4. Effect of administration
of combination of doxorubicin-docetaxel and combination of doxorubicin-docetaxel-pirolin
on activity of superoxide dismutase and plasma non-enzymatic antioxidant
capacity (NEAC) of rats bearing DMBA-induced mammary tumors: A:
SOD, B: NEAC. Each data point is presented as a median and lower-upper
quartile range. *p<0.05 in relation to untreated animals. Untreated
- rats with DMBA-induced mammary tumors injected with a vehicle for investigated
compounds (5% glucose); DOX-DTX (l) - rats treated with combination
of lower doses of DOX (2.5 mg/kg b.w.) and DTX (3.75 mg/kg b.w.); DOX-DTX
(l)+PL - rats treated with combination of lower doses of DOX (2.5
mg/kg b.w.) and DTX (3.75 mg/kg b.w.) with addition of pirolin (10 mg/kg
b.w.); DOX-DTX (h) - rats treated with combination of higher doses
of DOX (5 mg/kg b.w.) and DTX (7.5 mg/kg b.w.); DOX-DTX (h)+PL
- rats treated with combination of higher doses of DOX (5 mg/kg b.w.)
and DTX (7.5 mg/kg b.w.) with addition of pirolin (10 mg/kg b.w.). |
Although doxorubicin and docetaxel, independently on the dose, generated considerable increase in NEAC, higher value of this parameter was observed in rats receiving lower doses of anticancer drugs. This effect was further enhanced by the addition of pirolin.
4. Oxidative damage to lipids
4.1 Estimation of hydroperoxides
The amount of hydroperoxides estimated in untreated controls was equal to 0.4 (0.29; 0.59) nmol/mg protein. It became significantly elevated (about 2.5-fold) in rats administered with lower doses of anticancer drugs. Strikingly greater changes (about a 7-fold rise in relation to untreated controls) were found in rats treated with higher doses of DOX-DTX.
Inclusion of PL to chemotherapy employing lower drug doses enhanced DOX-DTX
effect and led to a further augmentation of hydroperoxides. At the same time
pirolin injected concurrently with higher doses of DOX-DTX did not show any
significant effects (
Fig. 5, 5A).
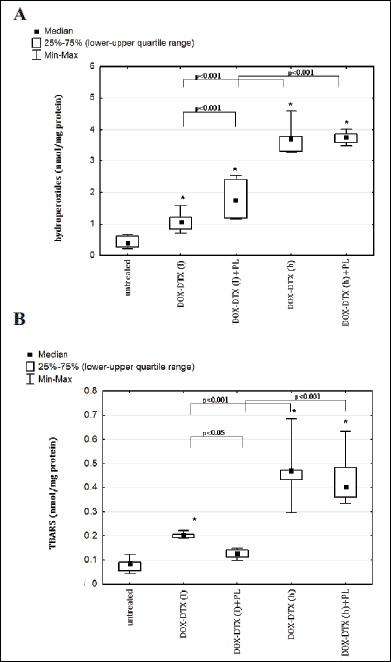 |
Fig. 5. Effect of administration
of combination of doxorubicin-docetaxel and combination of doxorubicin-docetaxel-pirolin
on lipid peroxidation in blood plasma of rats bearing DMBA-induced mammary
tumors: A: hydroperoxides, B: TBARS. Each data point is
presented as a median and lower-upper quartile range. *p<0.05 in relation
to untreated animals. Untreated - rats with DMBA-induced mammary tumors
injected with a vehicle for investigated compounds (5% glucose); DOX-DTX
(l) - rats treated with combination of lower doses of DOX (2.5 mg/kg
b.w.), DTX (3.75 mg/kg b.w.); DOX-DTX (l)+PL - rats treated with
combination of lower doses of DOX (2.5 mg/kg b.w.) and DTX (3.75 mg/kg
b.w.) and pirolin (10 mg/kg b.w.); DOX-DTX (h) - rats treated with
combination of higher doses of DOX (5 mg/kg b.w.) and DTX (7.5 mg/kg b.w.);
DOX-DTX (h)+PL - rats treated with combination of higher doses
of DOX (5 mg/kg b.w.), DTX (7.5 mg/kg b.w.) and pirolin (10 mg/kg b.w.). |
4.2 Measurement of thiobarbituric acid-reactive substances (TBARS)
Lipid peroxidation in blood plasma was further evidenced by the formation of
TBARS (
Fig. 5, 5B). Higher level of TBARS was observed in rats receiving
drugs alone. It is worth mentioning that PL showed partial protection against
lipid peroxidation induced by lower doses of DOX and DTX. Nitroxide was ineffective
in protection of plasma lipids against prooxidative activity of these anticancer
drugs when they were applied at higher doses.
DISCUSSION
Chemotherapy is an essential step of cancer treatment. An optimal protocol usually employs two or more drugs, since such treatment is correlated with better therapeutic efficacy. On the other hand, polychemotherapy may show greater toxicity toward non-targeted tissues. Numerous undesirable effects such as fatal cardiomyopathy and free radical formation with depletion of detoxifying enzymes leading to significant oxidative stress have been found for combination therapy involving two or more drugs,
e.g. doxorubicin and taxane (34).
Doxorubicin can evoke significant oxidative stress (35) and such a mechanism of docetaxel activity has also been suggested (36). Doxorubicin quinone moiety easily undergoes one electron redox cycling with molecular oxygen (37). The resulting compound is superoxide, which can produce highly reactive oxygen species such as hydroxyl radical or peroxynitrite (38). Doxorubicin can also form complexes with iron to produce ROS (39). Moreover, taxanes promote conversion of doxorubicin to the toxic metabolite doxorubicinol and thus can enhance toxicity of anthracycline (40).
Our study was designed to support the hypothesis that combined doxorubicin-docetaxel chemotherapy causes oxidative damage in the blood plasma that can be attenuated by a synthetic putative antioxidant pirolin (pyrroline nitroxyl derivative), administered in conjunction with these drugs. Oxidative stress in the plasma is potentially dangerous to different organs, because of impairment in the proteins, including transporters of hormones, nutrients and other substances that may be insufficiently supplied. Therefore, the application of compounds with antioxidant properties is of the highest interest. Based upon the fact that nitroxides present assortment of antioxidative properties, we hypothesized that inclusion of pirolin in DOX-DTX treatment may ensure protection against oxidative damage to plasma constituents.
The efficacy of pirolin as chemoprotector was evaluated on the basis of changes in the major biomarkers of oxidative damage to proteins and lipids such as carbonyl and thiol groups, hydroperoxides and low molecular weight end products TBARS. Amount of carbonyl and thiol groups in blood plasma are considered as useful biomarkers of oxidative protein damage and an increase in carbonyl groups has been shown in a variety of diseases and processes (41, 42). In contrast, either an increase or a decrease in the amount of -SH groups has been reported. Elevation of -SH group content has been explained on the basis of their defensive role and ability to undergo redox reversible thiol-dithiols exchange in order to mediate the oxidant-induced stress (43). In turn, a decrease in the amount of thiol groups can suggest depletion of thiols in conditions of extensive oxidative stress (44).
Since plasma antioxidant system comprises of both enzymatic and non-enzymatic mechanisms, we also measured total non-enzymatic antioxidant capacity of plasma and activity of SOD as the first line of defense against superoxide radicals.
Changes in oxidative stress-related parameters in untreated rats with 7,12-dimethylbenz(a)anthracene-induced tumors
We have observed an increase in the amount of both carbonyl and thiol groups in blood plasma of rats with DMBA-induced mammary tumors that have not been treated with any of the investigated compounds. It has been shown previously that under oxidizing conditions, associated with persistent stress, the amount of low-molecular-weight thiols may increase due to the release of cellular glutathione (45). An increase in the content of thiols may be also due to the activity of thioredoxin, which preserves the reduced state of protein -SH groups (46). In untreated rats with DMBA-induced tumors we also observed a significant increase in lipid peroxidation products with a concomitant decrease in SOD activity, which confirm data of other authors showing that oxidative stress is associated with the processes of carcinogenesis and tumor development (47). On the other hand an increase of NEAC in the plasma of DMBA-treated rats found in our study could suggest some pathological changes in the renal or hepatic physiology. It has been found, for example, that in patients with chronic renal failure the level of reducing potential of plasma was significantly elevated (48, 49). It is linked to an increase of uric acid, which amount is of the utmost importance for the plasma total antioxidant activity. An increase of this compound has been found after DMBA administration in rats (50).
Effects of pirolin administered as a single agent on rats bearing 7,12-dimethylbenz(a)anthracene-induced tumors
Considering different data on dual (antioxidant and pro-oxidant) properties of nitroxides, dependently on the model of study and experimental conditions (21, 51), we additionally investigated the effect of the mode of pirolin administration on oxidative status of blood plasma.
Two protocols of treatment with pirolin have been applied. Employment of protocol A resulted in an increase in the amount of protein carbonyl groups while protocol B led to reduction of their amount to the level of healthy controls.
Treatment with pirolin induced an increase in the TBARS level (about 2-fold greater for protocol B), indicating that this nitroxide, despite its potential protective effect on protein, can provoke lipid peroxidation. Parallel, about 2-fold elevation in the hydroperoxides was present when pirolin was applied according to protocol A. Protocol B caused opposite effect - about a 2-fold decrease of hydroperoxides which reached the level found in healthy controls. The observed discrepancy in these results may be interpreted by the nature of hydroperoxides. They are the primary and unstable products of lipid peroxidation process and may undergo secondary reactions giving the end products of peroxidation - TBARS. Indeed, we observed significantly higher level of TBARS in the group of rats treated with PL according to protocol B. Our and other studies suggest that it cannot be concluded on the basis of the amount of hydroperoxides only about the extent of lipid damage (52). The selective effect of pirolin on lipids also shows that these biomolecules are the most vulnerable to the attack of reactive oxygen species (53). We did not find any significant changes in both the activity of SOD and NEAC in respect to untreated rats after administration of PL according to protocol B, while an increase in these activities was evident at the treatment using protocol A. Taken together, these results suggest both antioxidative and pro-oxidative effects of pirolin
in vivo depending on the cumulative dose of the nitroxide and the regimen of treatment.
Dual character of nitroxyl derivatives has been described in many papers (51, 54). The pro-oxidative properties of pirolin found in our study could be attributed to the ability of nitroxides to shuffle between three oxidation states and formation of strongly oxidizing agent,
i.e. oxoammonium cations. These oxidized forms of nitroxides can mediate damage to the biomolecules in some conditions (55). It has been shown previously that nitroxides may also evoke oxidative stress depending on the experimental model (56). In human cancer cells, nitroxide TEMPO caused an increase in hydrogen peroxide production (57). Thus, it may be possible that a similar effect of nitroxide appeared in our study.
Effects of doxorubicin-docetaxel chemotherapy
Combined DOX-DTX chemotherapy generated oxidative damage to plasma proteins only when the drugs have been used at higher doses. In this group of animals about a 2-fold increase in protein carbonyl groups with a concomitant depletion of blood plasma thiols in respect to untreated controls was found. Lower drug doses caused a decrease in the amount of carbonyl groups, which may suggest efficient antioxidant activity of thiols allowing restoration of oxidatively damaged molecules. Thiol pool drastically decreased after treatment with both higher and lower drug doses. Free radicals generated by DOX can also promote oxidation of thiol groups (58). The observed reduction of the amount of -SH groups may also confirm the effectiveness of chemotherapy,
i.e. drugs may provoke an adaptive response as an increase in nonprotein antioxidant capacity. Such effect was observed in cancerous patients (59).
Combined DOX-DTX treatment induced a dose-dependent increase in lipid peroxidation. In our study rats treated with chemotherapy employing higher drug doses displayed significant higher level (2-3-fold) of both lipid peroxidation biomarkers compared to the chemotherapy with lower drug doses.
Both lower and higher drug doses caused a significant increase of plasma antioxidant capacity and SOD activity. Non-enzymatic antioxidant capacity (NEAC) or total antioxidant capacity of plasma (TCP) depends on concentration of several low molecular weight antioxidants such as ascorbic and uric acids, a-tocopherol, bilirubin, thiols and other undefined substances. Observed elevation of NEAC after DOX-DTX administration in our study is in agreement with findings reported by other authors, where an increase of bilirubin (approx. 4-fold) (60) and uric acid (approx. 2-fold) was found after DOX treatment (61). Other studies indicated higher total antioxidant status (TAS) in blood plasma of patients with chronic kidney disease, which was probably influenced by an increase of uric acid level (62). In our study, doxorubicin and docetaxel might induce damage of renal and hepatic functions, probably reflected in increased bilirubin and uric acid plasma levels. On the other hand, an increase of NEAC and SOD activity can be also a consequence of the response of an organism to oxidative stress induced by anticancer drugs. This outcome suggests that significant oxidative injury caused by high DOX-DTX doses in our experimental conditions could not be counterbalanced by antioxidant defense systems. Our results also confirm previous reports on doxorubicin and docetaxel related toxicity to lipids (63, 64).
In summary, the polychemotherapy employing doxorubicin and docetaxel results in the modification of biochemical parameters. The observed increase in the lipid and protein oxidation markers after DOX-DTX administration strongly indicates the role of chemotherapy in induction of oxidative stress, which may account for some of the side effects of cancer treatment. The finding that higher doses of DOX and DTX evoked more insult to the biomolecules is in line with the reports showing that higher cumulative dose of drugs results in more non-targeted tissue damage (63, 64).
Effects of pirolin on oxidative stress induced by doxorubicin-docetaxel chemotherapy
Some antioxidant activity of pirolin was observed in relation to blood plasma proteins when a nitroxide was used in conjunction with higher doses of DOX and DTX. About 25% decrease in protein carbonyls in rats concurrently treated with DOX, DTX and PL was found in respect to rats receiving combination of both drugs without addition of pirolin. This effect of a nitroxide was not seen in the group of rats treated with lower drug doses.
Combination of PL with lower doses of drugs elicited greater decrease of TBARS
compared with the group of animals administered with higher doses of DOX-DTX.
However, the level of these products was still far above control. No such antioxidant
properties of PL were observed in regard to hydroperoxides. Conversely, addition
of a nitroxide to the lower doses of DOX-DTX caused a further increase in hydroperoxides.
Pirolin was relatively inefficient in preservation of lipids in the case of
the higher doses of DOX-DTX, which may suggest that its concentration was too
low to counterbalance induced oxidative damage to these macromolecules. Lack
of pirolin protection may be also explained by the free radical nature of nitroxides.
It is possible that the conditions created in the plasma after chemotherapy
promoted reduction of nitroxides beyond the stage of hydroxyloamines thus affecting
antioxidant properties of these compounds. There is, for example, a report presenting
that harmful glutathionyl radical GS
• reacts with
nitroxides to provide secondary amines (66).
Addition of PL to DOX-DTX evoked a significant increase in SOD activity only when lower drug doses were used.
The results regarding the action of a nitroxide administered together with DOX-DTX chemotherapy suggest that pirolin could be a modulator of oxidative stress generated by these drugs. Its inclusion in DOX-DTX chemotherapy can partially protect blood plasma proteins and lipids against oxidative damage generated by these drugs. Further investigation is needed in order to establish optimal doses and treatment regimen to ensure best protection. It should be underlined that equally carcinogen and antioxidant/prooxidant effects
in vivo are strongly related to changes in expression of numerous genes encoding proteins, which are involved in various biological processes. This may result in deregulation of many important pathways. Some of them might lead to tumor development and progression and disturbances in oxidative status of cells. Recently, the dog-specific cDNA microarray was used by Rao
et al. (67) to investigate altered gene expressions in progestin-induced canine mammary hyperplasia (CMH) and in spontaneous canine mammary tumors (CMC). They showed that main genes, which expression were altered in CMH were those involved in cell proliferation and process of cell migration. Upregulation of expression was observed in the case of genes, which were involved in stimulation of cell proliferation and inhibition of apotosis, whereas downregulation of expression was found in the case of genes encoding positive regulators or factors responsible for the impediment of growth. Moreover, gene encoding carbonyl reductase-3 was upregulated in both, progestin-induced canine mammary hyperplasia (CMH) and in spontaneous canine mammary tumors (CMC). It should be mentioned that the product of this gene is involved in doxorubicin conversion into cardiotoxic alcoholic metabolites and its SNP mutation is also linked to the risk of cardiomyopathy development after doxorubicin treatment (68).
Other mechanism of antioxidants action is their effect on DNA synthesis, damage and repair. Comparison of cellular and tissue transcriptional profiles in canine mammary tumors revealed significant changes in expression of genes involved in signal transduction, protein, nucleotide and nucleic acid metabolism (69). Notably, the gene expression profiles in cell cultures derived from tumors of two different origins (chondrosarcoma and adenocarcinoma) were very much alike to that in parental tumors
in vivo.
The result obtained in pancreatic PANC-1 carcinoma cells by Sliwinska
et al. (70) after their treatment with gliclazide, a second-generation sulfonylurea with potential antioxidant properties, suggest that the protective effect of this compound might be partially connected with stimulation of DNA repair
e.g. expression of NHEJ genes. We might, therefore, speculate that the effect of the nitroxide observed in our study could be the outcome of both its direct free radical scavenging properties and induced differential gene expression.
Acknowledgements:
The authors would like to thank Prof. dr hab. Cezary Watala for his excellent
support and valuable discussion in statistical analysis of results.
Financial support for this work was provided by Grant N 401 2337 33 of Ministry
of Science and Higher Education (Poland).
Conflict of interests: None declared.
REFERENCES
- Radaideh SM, Sledge GW. Taxane vs. taxane: is the duel at an end? A commentary
on a phase-III trial of doxorubicin and docetaxel versus doxorubicin and
paclitaxel in metastatic breast cancer: results of the ERASME 3 study. Breast
Cancer Res Treat 2008; 111: 203-208.
- Box VG. The intercalation of DNA double helices with doxorubicin and nogalamycin.
J Mol Graph Model 2007; 26: 14-19.
- Burden DA, Osheroff N. Mechanism of action of eukaryotic topoisomerase
II and drugs targeted to the enzyme. Biochim Biophys Acta 1998; 1400: 139-154.
- Koceva-Chyla A, Jedrzejczak M, Skierski J, Kania K, Jozwiak Z. Mechanisms
of induction of apoptosis by anthraquinone anticancer drugs aclarubicin
and mitoxantrone in comparison with doxorubicin: relation to drug cytotoxicity
and caspase-3 activation. Apoptosis 2005; 10: 1497-1514.
- Swift LP, Rephaeli A, Nudelman A, Phillips DR, Cutts SM. Doxorubicin-DNA
adducts induce a non-topoisomerase II-mediated form of cell death. Cancer
Res 2006; 66: 4863-4871.
- Wang S, Konorev EA, Kotamraju S, Joseph J, Kalivendi S, Kalyanaraman B.
Doxorubicin induces apoptosis in normal and tumor cells via distinctly
different mechanisms. intermediacy of H2O2- and p53-dependent pathways.
J Biol Chem 2004; 279: 25535-25543.
- Morse DL, Gray H, Payne CM, Gillies RJ. Docetaxel induces cell death through
mitotic catastrophe in human breast cancer cells. Mol Cancer Ther 2005;
4: 1495-1504.
- Cao D, Qiao B, Ge Z, Yuan Y. Amplification loop cascade for increasing
caspase activity induced by docetaxel. J Cell Biochem 2005; 96: 810-820.
- Peng X, Chen B, Lim CC, Sawyer DB. The cardiotoxicology of anthracycline
chemotherapeutics: translating molecular mechanism into preventative medicine.
Mol Interv 2005; 5: 163-171.
- Salvatorelli E, Menna P, Cascegna S, et al. Paclitaxel and docetaxel
stimulation of doxorubicinol formation in the human heart: implications
for cardiotoxicity of doxorubicin-taxane chemotherapies. J Pharmacol Exp
Ther 2006; 318: 424-433.
- Shimoyama M, Murata Y, Sumi K, Hamazoe R, Komuro I. Docetaxel induced
cardiotoxicity. Heart 2001; 86: 217-220.
- Mir O, Alexandre J, Tran A, et al. Relationship between GSTP1 Ile(105)Val
polymorphism and docetaxel-induced peripheral neuropathy: clinical evidence
of a role of oxidative stress in taxane toxicity. Ann Oncol 2009; 20: 736-740.
- Damiani E, Kalinska B, Canapa A, et al. The effects of nitroxide
radicals on oxidative DNA damage. Free Radic Biol Med 2000; 28: 1257-1265.
- Zhang R, Goldstein S, Samuni A. Kinetics of superoxide-induced exchange
among nitroxide antioxidants and their oxidized and reduced forms. Free
Radic Biol Med 1999; 26: 1245-1252.
- Samuni A, Goldstein S, Russo A, Mitchell JB, Krishna MC, Neta P. Kinetics
and mechanism of hydroxyl radical and OH-adduct radical reactions with nitroxides
and with their hydroxylamines. J Am Chem Soc 2002; 124: 8719-8724.
- Offer T, Mohsen M, Samuni A. An SOD-mimicry mechanism underlies the role
of nitroxides in protecting papain from oxidative inactivation. Free Radic
Biol Med 1998; 25: 832-838.
- Krishna MC, Samuni A, Taira J, Goldstein S, Mitchell JB, Russo A. Stimulation
by nitroxides of catalase-like activity of hemeproteins. Kinetics and mechanism.
J Biol Chem 1996; 271: 26018-26025.
- Yamasaki T, Ito Y, Mito F, et al. Structural concept of nitroxide
as a lipid peroxidation inhibitor. J Org Chem 2011; 76: 4144-4148.
- Koceva-Chyla A, Gwozdzinski K, Kochman A, Stolarska A, Jozwiak Z. Effects
of pyrroline and pyrrolidine nitroxides on lipid peroxidation in heart tissue
of rats treated with doxorubicin. Cell Mol Biol Lett 2003; 8: 179-183.
- Koceva-Chyla A, Sokal A, Kania K, Gwozdzinski K, Jozwiak Z. The nitroxides
pirolin and pirolid protect the plasma membranes of rat cardiomyocytes against
damage induced by anthracyclines. Cell Mol Biol Lett 2003; 8: 171-177.
- Czepas J, Koceva-Chyla A, Gwozdzinski K, Jozwiak Z. Different effectiveness
of piperidine nitroxides against oxidative stress induced by doxorubicin
and hydrogen peroxide. Cell Biol Toxicol 2008; 24: 101-112.
- Chen Y, Jungsuwadee P, Vore M, Butterfield DA, St. Clair DK. Collateral
damage in cancer therapy: oxidative stress in nontargeted tissues. Mol Interv
2007; 7: 147-156.
- Rozantsev EG. Svobodnyje iminoksylnyje radicaly. Moscow: Chimia, 1970.
- Barros AC, Muranaka EN, Mori LJ, et al. Induction of experimental
mammary carcinogenesis in rats with 7,12-dimethylbenz(a)anthracene. Rev
Hosp Clin Fac Med Sao Paulo 2004; 59: 257-261.
- Whitsett T, Carpenter M, Lamartiniere CA. Resveratrol, but not EGCG, in
the diet suppresses DMBA-induced mammary cancer in rats. J Carcinog 2006;
5: 15.
- Ellman GL. Tissue sulfhydryl groups. Arch Biochem Biophys 1959; 82: 70-77.
- Levine RL, Wehr N, Williams JA, Stadtman ER, Shacter E. Determination
of carbonyl groups in oxidized proteins. Meth Mol Biol 2000; 99: 15-24.
- Misra H, Fridovich I. The role of superoxide anion in the autooxidation
of epinephrine and a simple assay for superoxide dismutase. J Biol Chem
1972; 247: 3170-3175.
- Yamaguchi T, Takamura H, Matoba T, Terao J. HPLC method for evaluation
of the free radical-scavenging activity of foods by using 1,1-diphenyl-2-picrylhydrazyl.
Biosci Biotechnol Biochem 1998; 62: 1201-1204.
- Gay C, Gebicki JM. A critical evaluation of the effect of sorbitol on
the ferric-xylenol orange hydroperoxide assay. Anal Biochem 2000; 284: 217-220.
- Rice-Evans CA, Diplock AT, Symons MCR, Burdon RH, Knippenberg PH, (eds).
Techniques in Free Radicals Research. Amsterdam, London, Elsevier, 1991.
- Lowry OH, Rosebrough NG, Farr AL, Randall RJ. Protein measurement with
the folin phenol reagent. J Biol Chem 1951; 193: 265-275.
- Koceva-Chyla A, Sokal A, Kania K, Gwozdzinski K, Jozwiak Z. Protective
effect of nitroxides against damage to rat cardiomyocytes treated with doxorubicin.
In: Proceedings of XI Biennial Meeting of the Society for Free Radical Research
International, C Pasquier (ed.) Bologna, Monduzzi Editore, 2002, pp. 693-696.
- Minotti G, Saponiero A, Licata S, et al. Paclitaxel and docetaxel
enhance the metabolism of doxorubicin to toxic species in human myocardium.
Clin Cancer Res 2001; 7: 1511-1515.
- Aluise CD, Miriyala S, Noel T, et al. 2-Mercaptoethane sulfonate
prevents doxorubicin-induced plasma protein oxidation and TNF-
release: Implications for the reactive oxygen species-mediated mechanisms
of chemobrain. Free Radic Biol Med 2011; 50: 1630-1638.
- Mizumachi T, Suzuki S, Naito A, et al. Increased mitochondrial
DNA induces acquired docetaxel resistance in head and neck cancer cells.
Oncogene 2008; 27: 831-838.
- Gutierrez PL. The metabolism of quinone-containing alkylating agents:
free radical production and measurement. Front Biosci 2000; 5: D629-D638.
- Szabo C, Ischiropoulos H, Radi R. Peroxynitrite: biochemistry, pathophysiology
and development of therapeutics. Nat Rev Drug Discov 2007; 6: 662-680.
- Kostoryz EL, Yourtee DM. Oxidative mutagenesis of doxorubicin-Fe(III)
complex. Mutat Res 2001; 490: 131-139.
- Gianni L, Vigano` L, Locatelli A, et al. Human pharmacokinetic
characterization and in vitro study of the interaction between doxorubicin
and paclitaxel in patients with breast cancer. J Clin Oncol 1997; 15: 1906-1915.
- Davies MJ, Fu S, Wang H, Dean RT. Stable markers of oxidant damage to
proteins and their application in the study of human disease. Free Radic
Biol Med 1997; 27: 1151-1163.
- Dalle-Donne I, Giustarini D, Colombo R, Rossi R, Milzani A. Protein carbonylation
in human diseases. Trends Mol Med 2003; 9: 169-176.
- Gilbert, HF. Molecular and cellular aspects of thiol-disulfide exchange.
Adv Enzymol Relat Areas Mol Biol 1990; 63: 69-172.
- Jonas CR, Puckett AB, Kurtz JC, et al. High-dose chemotherapy decreases
plasma glutatione (GSH) in patients undergoing bone marrow transplantion
(BMT). FASEB J 1997; 11: A649.
- Sies H, Akerboom TP. Glutathione (GSSG) efflux from cells and tissues.
Methods Enzymol 1984; 105: 445-451.
- Arner ES, Holmgren A. Physiological functions of thioredoxin and thioredoxin
reductase. Eur J Biochem 2000; 267: 6102-6109.
- Kumar B, Koul S, Khandrika L, Meacham RB, Koul HK. Oxidative stress is
inherent in prostate cancer cells and is required for aggressive phenotype.
Cancer Res 2008; 68: 1777-1785.
- Pieniazek A, Gwozdzinski K, Bujak S, Luciak M, Zbrug Z. Changes in reducing
ability of blond plasma in chronic renal failure patients during hemodialysis.
I: Proceedings of XI Biennial Meeting of the Society for Free Radical Research
International, C Pasquier (ed.) Bologna, Monduzzi Editore, 2002, pp. 693-696.
- Young IS. Measurement of total antioxidant capacity. J Clin Pathol 2001;
54: 339.
- Ozdemir I, Selamoglu Z, Ates B, Gok Y, Yilmaz I. Modulation of DMBA-induced
biochemical changes by organoselenium compounds in blood of rats. Indian
J Biochem Biophys 2007; 44: 257-259.
- Gariboldi MB, Rimoldi V, Supino R, Favini E, Monti E. The nitroxide Tempol
induces oxidative stress, p21WAF1/CIP1 and cell death in HL60 cells. Free
Radic Biol Med 2000; 29: 633-641.
- Niki E. Lipid peroxidation products as oxidative stress biomarkers. Biofactors
2008; 34: 171-180.
- Niki E, Yoshida Y, Saito Y, Noguchi N. Lipid peroxidation: mechanisms,
inhibition, and biological effects. Biochem Biophys Res Commun 2005; 338:
668-676.
- Koceva-Chyla A, Kochman A, Glebska J, Gwozdzinski K, Jozwiak Z, Metodiewa
D. Tempicol-3, a novel piperidine-N-noxide stable radical and antioxidant,
with low toxicity acts as apoptosis inducer and cell proliferation modifier
of Yoshida Sarcoma cells in vivo. Anticancer Res 2000; 20: 4611-4618.
- Dragutan I, Mehlhorn RJ. Modulation of oxidative damage by nitroxide free
radicals. Free Radic Res 2007; 41: 303-315.
- Offer T, Russo A, Samuni A. The pro-oxidative activity of SOD and nitroxide
SOD mimics. FASEB J 2000; 14: 1215-1223.
- Voest EE, van Faassen E, van Asbeck BS, Neijt JP, Marx JJ. Increased hydrogen
peroxide concentration in human tumor cells due to a nitroxide free radical.
Biochim Biophys Acta 1992; 1136: 113-118.
- Oliveira PJ, Santos MS, Wallace KB. Doxorubicin-induced thiol-dependent
alteration of cardiac mitochondrial permeability transition and respiration.
Biochemistry 2006; 71: 194-199.
- Di Giacomo C, Acquaviva R, Lanteri R, Licata F, Licata A, Vanella A. Nonproteic
antioxidant status in plasma of subjects with colon cancer. Exp Biol Med
(Maywood) 2003; 228: 525-528.
- Alshabanah OA, Hafez MM, Al-Harbi MM, et al. Doxorubicin toxicity
can be ameliorated during antioxidant L-carnitine supplementation. Oxid
Med Cell Longev 2010; 3: 428-433.
- Oz E, Ilhan MN. Effects of melatonin in reducing the toxic effects of
doxorubicin. Mol Cell Biochem 2006; 286: 11-15.
- Dounousi E, Papavasiliou E, Makedou A, et al. Oxidative stress
is progressively enhanced with advancing stages of CKD. Am J Kidney Dis
2006; 48: 752-760.
- Aluise CD, St Clair D, Vore M, Butterfield DA. in vivo amelioration
of adriamycin induced oxidative stress in plasma by gamma-glutamylcysteine
ethyl ester (GCEE). Cancer Lett 2009; 282: 25-29.
- Yang Z, Fong DW, Yin L, Wong Y, Huang W. Liposomes modulate docetaxel-induced
lipid oxidization and membrane damage in human hepatoma cells. J Liposome
Res 2009; 19: 122-130.
- Swain SM, Whaley FS, Ewer MS. Congestive heart failure in patients treated
with doxorubicin: a retrospective analysis of three trials. Cancer 2003;
97: 2869-2879.
- Borisenko GG, Martin I, Zhao Q, Amoscato AA, Kagan VE. Nitroxides scavenge
myeloperoxidase-catalyzed thiyl radicals in model systems and in cells.
J Am Chem Soc 2004; 126: 9221-9232.
- Rao NA, van Wolferen ME, Gracanin A, et al. Gene expression profiles
of progestin-induced canine mammary hyperplasia and spontaneous mammary
tumors. J Physiol Pharmacol 2009; 60(Suppl 1): 73-84.
- Fan L, Goh BC, Wong CI, et al. Genotype of human carbonyl reductase
CBR3 correlates with doxorubicin disposition and toxicity. Pharmacogenet
Genomics 2008; 18: 623-631.
- Pawlowski KM, Krol M, Majewska A, et al. Comparison of cellular
and tissue transcriptional profiles in canine mammary tumor. J Physiol Pharmacol
2009; 60(Suppl 1): 85-94.
- Sliwinska A, Sliwinski T, Kasznicki J, Drzewoski J. Effect of gliclazide
on nucleotide excision repair (NER) and non-homologous DNA end joining (NHEJ)
in normal and cancer cells. J Physiol Pharmacol 2010; 61: 347-53.