Brain serotonin (5-hydroxytryptamine, 5-HT)
system is involved in a wide spectrum of physiological processes including sleep,
cognition, sensory perception, motor activity, temperature regulation, appetite,
hormone secretion, nociception and sexual behavior (1). Among at least 15 subpopulations
of 5-HT receptors the 5-HT
7 receptor is the
latest subtype to be identified (2-4). A high density of 5-HT
7 receptors has
been identified in the thalamus, cortex, hippocampus, hypothalamus and raphe
nuclei (5-7). Many antidepressant drugs influence the turnover and metabolism
of 5-HT in the brain and dysfunctions of the serotonergic system are thought
to be involved in the pathomechanism of depressive disorders (8). The presence
of the 5-HT
7 receptor in limbic structures suggests its involvement in the pathophysiology
of mood disorders. This receptor has also been implicated in mood regulation,
circadian rhytmicity and sleep, the disturbances of which are evident in the
course of depressive disorders (9, 10). Our earlier study documented an attenuation
of the effects of the activation of rat hippocampal 5-HT
7 receptors after treatment
with the tricyclic antidepressant, imipramine, and the selective 5-HT reuptake
inhibitor, citalopram (11). In animal models, inactivation or blockade of the
5-HT
7 receptor has been shown to induce antidepressant-like behavior (12-14).
Recent studies have demonstrated a synergistic interaction between the specific
5-HT
7 receptor antagonist SB 269970 and several antidepressant drugs (15, 16).
Moreover, blockade of the 5-HT
7 receptor may produce faster antidepressant effects
than do contemporary antidepressant drugs (17). We have also demonstrated that
stress-induced modifications of basal glutamatergic transmission and long-term
potentiation in the frontal cortex were prevented by the 5-HT
7 receptor antagonist
(18). These findings support the hypothesis that the 5-HT
7 receptor can be considered
as a potential target for the action of antidepressant drugs.
The delayed onset of the therapeutic effects of antidepressants and the similar therapeutic efficacies of drugs with different primary pharmacological profiles suggest that adaptive mechanisms beyond monoaminergic modulation at synapses may be involved in the antidepressant actions. Growing evidence indicates that abnormalities in the excitatory amino acid transmission play not only an important role in the pathophysiology of mood disorders but also that a common mechanism of various antidepressant therapies may involve modifications in the function of brain glutamatergic system (19-22).
Abnormalities of several behavioral processes during depressive disorders suggest an involvement of the frontal cortex in the pathophysiology of depression and antidepressive drug action (23-25). We have previously shown that repeated administration of imipramine or citalopram, lasting for 2 weeks, resulted in a decrease in the amplitude of glutamate-mediated field potentials evoked in layer II/III of the rat frontal cortex by stimulation of underlying sites as well as in a reduction in the amplitude ratio of pharmacologically isolated NMDA to AMPA/kainate receptor-mediated components of the field potential (26). We have also demonstrated imipramine treatment-related decrease of spontaneous glutamate release from terminals synapsing onto layer II/III pyramidal cells in the frontal cortex (27). These results suggest that chronic treatment with antidepressants may attenuate glutamatergic transmission in rat cerebral cortex.
Because our previous study showed that serotonin, acting through 5-HT
7 receptors, exerts a complex modulatory influence on glutamate- and GABA-mediated synaptic transmission in the rat hippocampus (28), it is conceivable that the antidepressant-like effects of the 5-HT
7 receptor antagonist (12-14) might be related to its modulatory action on glutamatergic transmission, also in other brain regions. Therefore, in the present study we aimed at finding the effects of single and repeated administration 5-HT
7 antagonist SB269970 on glutamatergic neurons and excitatory synaptic transmission in the rat frontal cortex.
MATERIALS AND METHODS
Treatment of animals
Experimental procedures were approved by the Animal Care and Use Committee at
the Institute of Pharmacology, Polish Academy of Sciences, and were carried
out in accordance with the European Community guidelines and national law. Male
Wistar rats, weighing approx. 140 g at the beginning of the experiment, were
housed in groups on a controlled light/dark cycle (light: 7.00-19.00). Standard
food and tap water were available
ad libitum. The rats received SB 269970
i.p. (1.25 mg/kg, dissolved in 0.9% NaCl, volume: 1 ml/kg; purchased from Tocris)
either as a single injection or repeatedly for 14 days. In the former group
the administration of SB 269970 was preceded by 13 daily injections of a 0.9%
NaCl. The dose of SB 269970 was chosen on the basis of preliminary microdialysis
experiments demonstrating 5-HT efflux in the frontal cortex (unpublished). The
animals of the control group received 0.9% NaCl (1 mg/kg) once daily for 14
days.
Slice preparation
Brain slices were prepared 2 days after the last substance administration. Rats
were anesthetized with isoflurane, decapitated, their brains were quickly removed
and placed in ice-cold artificial cerebrospinal fluid (aCSF) containing (in
mM): 130 NaCl, 5 KCl, 2.5 CaCl
2, 1.3 MgSO
4,
1.25 KH
2PO
4,
26 NaHCO
3, 10 D-glucose, bubbled with a mixture
of 95% O
2 and 5% CO
2.
Frontal cortical slices (420 µm thick) were cut in the coronal plane using a
vibrating microtome (VT1000; Leica Microsystems). Slices were stored submerged
in aCSF at 32±0.5°C.
Whole-cell recording
A slice was placed in the recording chamber where it was submerged and superfused
at 3 ml/min with warm (32±0.5°C), modified aCSF containing (in mM): 132 NaCl,
2 KCl, 2.5 CaCl
2, 1.3 MgSO
4,
1.25 KH
2PO
4,
26 NaHCO
3, 10 D-glucose, bubbled with 95% O
2
- 5% CO
2. Neurons were visualized using Zeiss
Axioskop 2 upright microscope using Nomarski optics, a 40× water immersion lens
and an infrared camera (29). Patch pipettes were pulled from borosilicate glass
capillaries (Clark Electromedical Instruments) using Sutter Instrument P97 puller.
The pipette solution contained (in mM): 130 K-gluconate, 5 NaCl, 0.3 CaCl
2,
2 MgCl
2, 10 HEPES, 5 Na
2-ATP,
0.4 Na-GTP and 1 EGTA. Osmolarity and pH were adjusted to 290 mOsm and 7.2,
respectively. Pipettes had open tip resistance of approx. 6 M

.
Signals were recorded using the SEC 05LX amplifier (NPI), filtered at 2 kHz
and digitized at 20 kHz using Digidata 1322A interface and Clampex 9.2 software
(Molecular Devices). Layer II/III cells were sampled from sites located approx.
2 mm lateral to the midline and approx. 0.3 mm below the pial surface. Pyramidal
neurons were identified by morphological criteria (27) and by the characteristics
of the response to intracellular current pulses (
Fig. 1A).
Analysis of intrinsic excitability and spontaneous excitatory postsynaptic currents (sEPSCs)
Response characteristics of the recorded neurons were evaluated in the current
clamp mode with intracellular injections of rectangular current pulses (500
ms). To determine the relationship between the current and firing rate, the
number of spikes evoked by current steps of increasing amplitude (increments:
20 pA) were determined (30). The gain (slope) and firing threshold (measured
as a current extrapolated at zero firing rate) parameters were determined from
the straight lines fitted to raw data (
Fig. 1D), and averaged (
Fig.
1E, 1F).
Next, cells were voltage-clamped at -76 mV and sEPSCs were recorded for 8 min.
Spontaneous EPSCs were detected off-line and analyzed using Mini Analysis software
(Synaptosoft, USA). Data were accepted for analysis when the access resistance
ranged between 15 and 18 M

and it was stable (<25% change) during recording. Since the noise level of recordings
was in a range of 4-5 pA, the amplitude and the area thresholds for the detection
of an event were set to 7 pA and 25 fC, respectively, which maximized the correct
identification of sEPSCs. Recorded traces were visually inspected following
automated analysis to prevent false positive identification and false negative
rejection of events. In part of the experiments, 2 mM kynurenic acid (Sigma
Aldrich) was added to aCSF.
Statistical analysis was carried out using one-way ANOVA followed by the Dunnett's
post hoc test (SigmaPlot 12, Systat Software Inc.).
RESULTS
The cells included in the analysis exhibited a regular spiking firing pattern
in response to a depolarizing current pulse (
Fig. 1A). Basic membrane
properties did not differ significantly between the three experimental groups
(number of control neurons: n=37; number of cells in slices from rats receiving
single and repeated administration of SB 269970: n=38 and n=34, respectively;
Fig. 1B, 1C). None of the recorded neurons demonstrated spontaneous spiking
activity. Analysis of the relationship between injected current and firing rate
(
Fig. 1D, 1E, 1F) demonstrated that neither single nor repeated administration
of SB 269970 modified the intrinsic excitability of frontal cortex pyramidal
neurons.
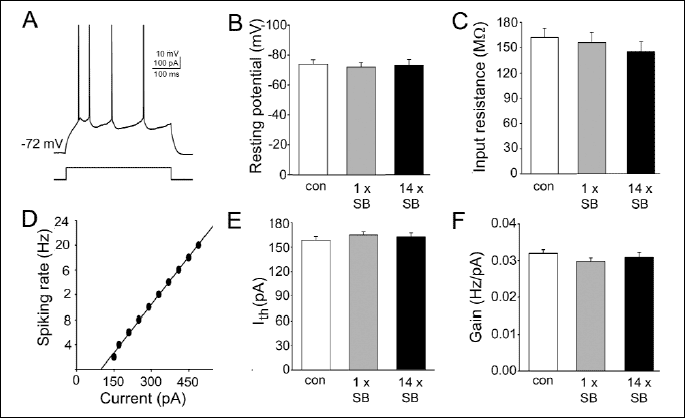 |
Fig.
1. Treatment with SB 269970 does not influence the intrinsic excitability
of pyramidal neurons. (A) A representative example of the response
(upper trace) to a depolarizing current pulse (lower trace), typical of
the layer II/III pyramidal cell. (B) Mean resting membrane potential
(±S.E.M.). In this and in the following panels: con, control neurons
(n=37); 1 x SB, cells (n=38) in slices from rats receiving single administration
of SB 269970, 14 x SB, cells (n=34) in slices from rats receiving repetitive
administration of SB 269970. (C) Mean input resistance (±S.E.M.).
(D) A representative example of the response characteristics of
a regular-spiking, pyramidal neuron. (E) Mean firing threshold
(±S.E.M.). (F) Mean gain (slope of spiking rate vs. injected
current relationship, ±S.E.M.). |
At the holding potential of -76 mV sEPSCs were recorded as inward currents (
Fig.
2A1, 2A2).
These sEPSCs could be blocked by 2 mM kunyrenic acid, a non-selective antagonist
at NMDA and AMPA/kainate receptors (
Fig. 2A3).
As illustrated in
Fig. 2B, in cells prepared from rats treated with SB
269970 for 14 days the mean frequency of sEPSCs was significantly lower (P<0.05)
in comparison to that in cells originating from control animals. Repeated treatment
with SB 269970 also resulted in a reduction in the mean amplitude of sEPSCs
(P<0.05). In contrast, single administration of SB269970 affected neither the
mean frequency nor the mean amplitude of sEPSCs (
Fig. 2B, 2C).
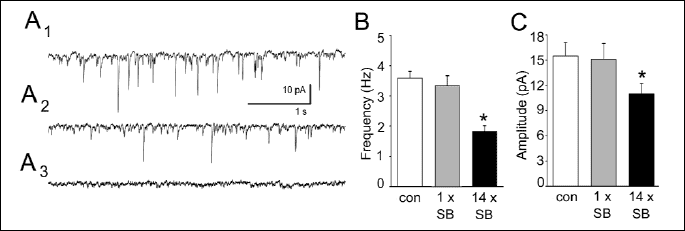 |
Fig.
2. Repeated administration of SB 269970 decreases the mean frequency
and the mean amplitude of sEPSCs. (A1)
A representative example of sEPSCs (downward deflections) recorded from
a control neuron. (A2) A representative
example of sEPSCs recorded from a neuron in a slice after repeated administration
of SB 269970. (A3) A representative
example of a recording in the presence of 2 mM kynurenic acid. (B) Mean
(±S.E.M.) frequency of sEPSCs in control neurons (con) and in neurons
originating from rats after single (1 x SB) and repeated (14 x SB) administration
of SB269970. * P<0.05 vs. control. (C) Mean (±
S.E.M.) amplitude of sEPSCs. Labels as in (B). |
DISCUSSION
The major finding of the present study is that repetitive administration of SB269970 induces a strong reduction in the mean frequency and a smaller decrease in the mean amplitude of spontaneous EPSCs recorded from cortical pyramidal neurons 2 days after the end of the treatment with this selective 5-HT
7 receptor antagonist.
As it was shown in our previous paper (27) most of the spontaneous EPSCs recorded from frontal cortical pyramidal neurons correspond to miniature EPSCs. Thus, the observed reduction in the frequency of sEPSCs is most likely related to presynaptic modifications of the mechanism of glutamate release (28). On the other hand, a decrease in the mean amplitude of sEPSCs may in principle result from pre- and/or postsynaptic modifications, involving a reduced glutamate content of synaptic vesicles within presynaptic terminals and/or alterations in postsynaptic reactivity to glutamate.
Some data show that serotonin regulates cortical function through modulation
of glutamatergic signaling. Frontal cortex receives a dense serotonergic input
from the dorsal raphe nucleus (DRN) (31). Serotonin, released from axon terminals
of the raphe neurons, has been shown to affect the action of several neurotransmitters
through heterosynaptic activation of 5-HT receptors located on neighboring axon
terminals (32). Heterosynaptic activation of 5-HT receptors on glutamatergic
neurons has been shown to inhibit or stimulate glutamate release in the cortex
via 5-HT
1A, 5-HT
1B
or 5-HT
2A receptors (33-35). Thus it is conceivable
that the decrease of glutamatergic transmission after chronic blockade of 5-HT
7
receptors, seen in the present study, occurs as a result of the activation of
other classes of 5-HT receptors by a prolonged, elevated level of 5-HT in the
cortex.
It has been shown that the blockade of 5-HT
7 receptors increases 5-HT raphe-hippocampus transmission (15). Enhancement of 5-HT transmission in such projection areas as the dorsal hippocampus after the administration of SB 269970 lasting 1 week has been reported by other researchers (17). So far only a few studies have examined the effect of SB-269970, administered alone or together with antidepressant drugs, on 5-HT release in the cortex. Wesolowska and Kowalska (36) have shown that SB-269970 increased the efflux of DA, NA, and 5-HT in the rat prefrontal cortex. On the other hand, some authors have found that SB-269970 significantly inhibits 5-HT efflux (37) and other investigators reported that inhibition of 5-HT release is likely to be mediated by 5-HT
7 receptor agonists (38). There is a possibility that the SB-269970-induced increase in 5-HT release could be mediated by the inhibition of 5-HT
7 receptors localized on GABAergic interneurons in the dorsal raphe nucleus (39). In a series of functional studies Roberts
et al. (37) and Glass
et al. (40) have proposed that 5-HT
7 receptors in the DRN are localized, among others, on GABAergic cells. We have demonstrated that the 5-HT
7 receptor-mediated increase in the sIPSCs frequency in hippocampal CA1 pyramidal cells is partially related to the activation of 5-HT
7 receptors located on GABAergic interneurons (28).
Another possible mechanism of the observed reduction of glutamatergic transmission after 14 days of SB269970 injections may potentially be related to the direct effects of the interaction between SB 269970 molecules and the 5-HT
7 receptor. It has been demonstrated that the activation of 5-HT
7 receptors increases neurite outgrowth in hippocampal neurons in the cell culture (41). Both Galpha(s) and Galpha(12) proteins can regulate cellular morphology by activating intracellular signaling cascades. These effects are produced either by modulation of cAMP concentration (42) or direct binding of Galpha(s) protein to the cytoskeleton (43). The downstream effectors of Galpha(12) protein, which mediate changes in the actin cytoskeleton, are members of the Rho family of small GTPases, including RhoA, Rac1 and Cdc42 (44). The major functional effects of this pathway, including actin reorganization and the formation of neurite-like protrusions, are mediated by the activation of Cdc42 (41). Since the activation of the 5-HT
7 receptor by Galpha(s) and Galpha(12) proteins stimulates glutamatergic synaptic transmission by a positive influence on a number of functional synapses, the prolonged blockade of the 5-HT
7 receptor might account for the observed reduction of glutamatergic transmission. However, it has recently been shown that 5-HT
7 receptor/Galpha(12) protein signaling pathway is functional only during early postnatal stages but not in adult animals (45), making this possibility less likely.
In conclusion, the results of the present study indicate for the first time that repeated administration of the 5-HT
7 receptor antagonist SB269970 results in a decrease of glutamatergic synaptic transmission in the rat frontal cortex. This phenomenon may have consequences for the development of new antidepressant therapies based on 5-HT
7 receptor antagonism.
Acknowledgements:
Supported by the Ministry of Science and Higher Education (Warsaw, Poland) grant
no. 0259/BP01/2010/38 and by the statutory funds from the Institute of Pharmacology,
Polish Academy of Sciences, Cracow, Poland.
Conflict of interests: None declared.
REFERENCES
- Jacobs BL, Azmitia EC. Structure and function of the brain serotonin system. Physiol Rev 1992; 72: 165-229.
- Bard JA, Zgombick J, Adham N, Vaysse P, Branchek TA, Weinshank RL. Cloning of a novel human serotonin receptor (5-HT7) positively linked to adenylate cyclase. J Biol Chem 1993; 268: 23422-23426.
- Ruat M, Traiffort E, Leurs R, et al. Molecular cloning, characterization, and localization of a high-affinity serotonin receptor (5-HT7) activating cAMP formation. Proc Natl Acad Sci USA 1993; 90: 8547-8551.
- Hoyer D, Hannon JP, Martin GR. Molecular, pharmacological and functional diversity of 5-HT receptors. Pharmacol Biochem Behav 2002; 71: 533-554.
- To ZP, Bonhaus DW, Eglen RM, Jakeman LB. Characterization and distribution of putative 5-HT7 receptors in guinea-pig brain. Br J Pharmacol 1995; 115: 107-116.
- Neumaier JF, Sexton TJ, Yracheta J, Diaz AM, Brownfield M. Localization
of 5-HT7 receptors in rat brain by immunocytochemistry,
in situ hybridization, and agonist stimulated cFos expression. J Chem Neuroanat
2001; 21: 63-73.
- Martin-Cora FJ, Pazos A. Autoradiographic distribution of 5-HT7 receptors
in the human brain using [3H]mesulergine:
comparison to other mammalian species. Br J Pharmacol 2004; 141: 92-104.
- Stahl SM. Mechanism of action of serotonin selective reuptake inhibitors. Serotonin receptors and pathways mediate therapeutic effects and side effects. J Affect Disord 1998; 51: 215-235.
- Hedlund PB, Sutcliffe JG. Functional, molecular and pharmacological advances
in 5-HT7 receptor research. Trends Pharmacol
Sci 2004; 25: 481-486.
- Thomas DR, Hagan JJ. 5-HT7 receptors. Curr Drug Targets CNS Neurol Disord 2004; 3: 81-90.
- Tokarski K, Zahorodna A, Bobula B, Grzegorzewska M, Pitra P, Hess G. Repeated
administration of citalopram and imipramine alters the responsiveness of
rat hippocampal circuitry to the activation of 5-HT7
receptors. Eur J Pharmacol 2005; 524: 60-66.
- Guscott M, Bristow LJ, Hadingham K, et al. Genetic knockout and pharmacological
blockade studies of the 5-HT7 receptor
suggest therapeutic potential in depression. Neuropharmacology 2005; 48:
492-502.
- Hedlund PB, Huitron-Resendiz S, Henriksen SJ, Sutcliffe JG. 5-HT7
receptor inhibition and inactivation induce antidepressantlike behavior
and sleep pattern. Biol Psychiatry 2005; 58: 831-837.
- Wesolowska A, Nikiforuk A, Stachowicz K, Tatarczynska E. Effect of the
selective 5-HT(7) receptor antagonist SB
269970 in animal models of anxiety and depression. Neuropharmacology 2006;
51: 578-586.
- Bonaventure P, Kelly L, Aluisio L, et al. Selective blockade of 5-hydroxytryptamine
(5-HT)7 receptors enhances 5-HT transmission,
antidepressant-like behavior, and rapid eye movement sleep suppression induced
by citalopram in rodents. J Pharmacol Exp Ther 2007; 321: 690-698.
- Wesolowska A, Tatarczynska E, Nikiforuk A, Chojnacka-Wojcik E. Enhancement
of the anti-immobility action of antidepressants by a selective 5-HT7
receptor antagonist in the forced swimming test in mice. Eur J Pharmacol
2007; 555: 43-47.
- Mnie-Filali O, Faure C, Lambas-Senas L, et al. Pharmacological blockade
of 5-HT7 receptors as a putative fast acting
antidepressant strategy. Neuropsychopharmacology 2011; 36: 1275-1288.
- Tokarski K, Bobula B, Kusek M, Hess G. The 5-HT7 receptor antagonist SB 269970 counteracts restraint stress-induced attenuation of long-term potentiation in rat frontal cortex. J Physiol Pharmacol 2011; 62: 663-667.
- Paul A, Skolnick P. Glutamate and depression: clinical and preclinical studies. Ann NY Acad Sci 2003; 1003: 250-272.
- Kugaya A, Sanacora G. Beyond monoamines: glutamatergic function in mood disorders. CNS Spectr 2005; 10: 808-819.
- Palucha A, Pilc A. The involvement of glutamate in the pathophysiology of depression. Drug News Perspect 2005; 18: 262-268.
- Schechter LE, Ring RH, Beyer CE, et al. Innovative approaches for the development of antidepressant drugs: current and future strategies. NeuroRx 2005; 5: 590-611.
- Manji HK, Drevets WC, Charney DS. The cellular neurobiology of depression. Nat Med 2001; 7: 541-547.
- Keedwell PA, Andrew C, Williams SC, Brammer MJ, Phillips ML. The neural correlates of anhedonia in major depressive disorder. Biol Psychiatry 2005; 58: 843-853.
- Krishnan V, Nestler EJ. Molecular neurobiology of depression. Nature 2008; 455: 894-902.
- Bobula B, Tokarski K, Hess G. Repeated administration of antidepressants decreases field potentials in rat frontal cortex. Neuroscience 2003; 120: 765-769.
- Tokarski K, Bobula B, Wabno J, Hess G. Repeated administration of imipramine attenuates glutamatergic transmission in rat frontal cortex. Neuroscience 2008; 153: 789-795.
- Tokarski K, Kusek M, Hess G. 5-HT7 receptors
modulate GABAergic transmission in rat hippocampal CA1 area. J Physiol Pharmacol
2011; 62: 535-540.
- Tokarski K, Zahorodna A, Bobula B, Hess G. 5-HT7
receptors increase the excitability of rat hippocampal CA1 pyramidal neurons.
Brain Res 2003; 993: 230-234.
- Bekisz M, Garkun Y, Wabno J, Hess G, Wrobel A, Kossut M. Increased excitability of cortical neurons induced by associative learning: an ex vivo study. Eur J Neurosci 2010; 32: 1715-1725.
- O'Hearn E, Molliver ME. Organization of raphe-cortical projections in rat: a quantitive retrograde study. Brain Res Bull 1984; 13: 709-726.
- Fink KB, Gothert M. 5-HT receptor regulation of neurotransmitter release. Pharmacol Rev 2007; 59: 360-417.
- Calcagno E, Carli M, Invernizzi RW. The 5-HT1A
receptor agonist 8-OH-DPAT prevents prefrontocortical glutamate and serotonin
release in response to blockade of cortical NMDA receptors. J Neurochem
2006; 96: 853-860.
- Golembiowska K, Dziubina A. Inhibition of amino acid release by 5-HT1B
receptor agonist in the rat prefrontal cortex. Pol J Pharmacol 2002; 54:
625-631.
- Maura G, Marcoli M, Tortarolo M, Andrioli, M. Raiteri M. Glutamate release in human cerebral cortex and its modulation by 5-hydroxytryptamine acting at h 5-HT1D receptors. Br J Pharmacol 1998; 123: 45-50.
- Wesolowska A, Kowalska M. Influence of serotonin 5-HT7
receptor blockade on the behavioral and neurochemical effects of imipramine
in rats. Pharmacol Rep 2008; 60: 464-474.
- Roberts C, Thomas DR, Bate ST, Kew JN. GABAergic modulation of 5-HT7 receptor-mediated effects on 5-HT efflux in the guinea-pig dorsal raphe nucleus. Neuropharmacology 2004; 46: 935-941.
- Harsing LG. The pharmacology of the neurochemical transmission in the midbrain raphe nuclei of the rat. Curr Neuropharmacol 2006; 4: 313-339.
- Duncan MJ, Temel S, Jennes L. Localization of serotonin 5-HT7 receptor immunoreactivity in the rat brain. Soc Neurosci Abstr 2001; 380: 18.
- Glass JD, Grossman GH, Farnbauch L, DiNardo L. Midbrain raphe modulation of nonphotic circadian clock resetting and 5-HT release in the mammalian suprachiasmatic nucleus. J Neurosci 2003; 23: 7451-7460.
- Kvachnina E, Liu G, Dityatev A, et al. 5-HT7
receptor is coupled to G alpha subunits of heterotrimeric G12-protein to
regulate gene transcription and neuronal morphology. J Neurosci 2005; 25:
7821-7830.
- Corset V, Nguyen-Ba-Charvet KT, Forcet C, Moyse E, Chedotal A, Mehlen
P. Netrin-1-mediated axon outgrowth and cAMP production requires interaction
with adenosine A2b receptor. Nature 2000;
407: 747-750.
- Yu JZ, Dave RH, Allen JA, Sarma T, Rasenick MM. Cytosolic G(alpha)s acts as an intracellular messenger to increase microtubule dynamics and promote neurite outgrowth. J Biol Chem 2009; 284: 10462-10472.
- Jaffe AB, Hall A. Rho GTPases: biochemistry and biology. Annu Rev Cell Dev Biol 2005; 21: 247-269.
- Kobe F, Guseva D, Jensen TP, et al. 5-HT7R/G12
signaling regulates neuronal morphology and function in an age-dependent
manner. J Neurosci 2012; 32: 2915-2930.