Peroxisome proliterator-activated receptors
(PPARs) are ligand-activated transcription factors belonging to the large superfamily
of nuclear receptors. Their endogenous agonists include a variety of long-chain
fatty acids and fatty acid derivatives such as prostaglandins and leukotrienes.
There are three major PPAR isoforms termed

,

and

which all form heterodimers with retinoid X receptor (RXR) and bind to PPAR
responsive element (PPRE) found in the promoter region of various genes involved
in lipid metabolism and energy homeostasis (17). PPAR

is expressed at a high level in tissues exhibiting high rates of fatty acid
ß-oxidation including liver, heart and kidney (12). In the recent years,
the fibrate class of hypolipidemic drugs which is widely used in patients with
metabolic syndrome has been shown to function through direct PPAR

activation (14).
PPAR

plays a pivotal
role in the transcriptional regulation of lipid metabolism in the heart.
In
vitro studies on isolated rodent cardiomyocytes showed that activation of
this receptor induces expression of a number of genes encoding proteins involved
in virtually all steps of fatty acid metabolism. These steps include: fatty
acid uptake (fatty acid transport protein, fatty acid translocase/CD36) and
activation (acyl-CoA synthetase), transport of acyl-CoA to mitochondrial matrix
(carnitine palmitoyltransferase (CPT) 1 and 2), mitochondrial ß-oxidation
(short, medium, long and very long-chain acyl-CoA dehydrogenases, short-chain
3-hydroxyacyl-CoA dehydrogenase, 3-ketoacyl-CoA thiolase) and peroxisomal b-oxidation
(acyl-CoA oxidase) (4). In addition, PPAR

activation induces expression of malonyl-CoA decarboxylase which is the enzyme
responsible for degradation of malonyl-CoA – a competitive inhibitor of CPT1
playing an important role in the regulation of mitochondrial fatty acid oxidation
in the heart (5). Consistently, PPAR

knockout mice exhibit considerably lower myocardial fatty acid oxidation rate
and expression of related enzymes (33). The heart of these animals is also unable
to adapt to increased fatty acid availability resulting from fasting or high-fat
feeding (11, 18). On the other hand, cardiac-specific overexpression of PPAR

leads to increased fatty acid utilization in the heart (15).
Over the last decade the role of PPAR

in the regulation of myocardial lipid metabolism was intensively studied. However, the vast majority of available papers focused on changes in expression of PPAR

-regulated genes and there is very few data on the effect of PPAR

agonists on the content and composition of myocardial lipids. Therefore, considering the importance of lipids in the membrane function and cellular signaling, our main aim was to examine effects of selective PPAR

agonist – WY-14643 on the content and fatty acid composition of major lipid classes in the rat heart.
MATERIALS AND METHODS
Animals and study design
The investigation was approved by the Ethical Committee for Animal Experiments
at the Medical University of Bialystok. Male Wistar rats (200-240g) were housed
in separate cages under controlled conditions (22°C ± 2, 12h light/12h dark
cycle) with unlimited access to water. The animals were divided into two groups:
1) fed ad libitum on a standard laboratory rat chow (Agropol, Motycz, Poland)
containing 2.8% of fat by weight and 2) fed for three weeks on a high-fat diet
containing 33.9% of fat (sunflower oil) by weight, prepared as described by
Pascoe and Storlien (28). The high-fat diet was given daily in the amount providing
a number of calories corresponding to the previously determined average energy
intake in the group of rats fed on the standard chow (82 kcal per animal per
day). The fatty acid composition of both diets is presented in
Table 1.
Each group was further divided into two subgroups: a) control and b) treated
daily for two weeks with a selective PPAR

agonist – WY-14643 (Cayman Chemical) in a dose of 3 mg/kg of body weight, starting
from the second week of the experiment. The drug was suspended in 0.5% methylcellulose
and administrated by an oral gavage.
Table 1. Fatty acid composition of the experimental diets. |
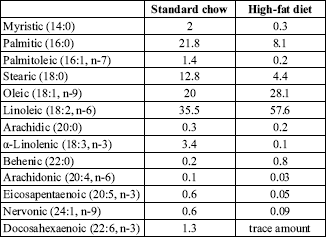 |
Fatty acid composition of the experimental diets was determined by means of gas-liquid chromatography. All values are expressed as mol %. |
The animals were anaesthetized by intraperitoneal injection of pentobarbital in a dose of 80 mg/kg of body weight. Samples of the left ventricle were excised, cleaned of the blood and immediately freeze-clamped with aluminum tongs precooled in liquid nitrogen and then stored at -80°C until analysis. All samples were collected between 8 and 10 am.
Content of myocardial lipids
The samples of the myocardium were pulverized in an aluminum mortar precooled in liquid nitrogen. The powder was then transferred to a tube containing ice-cold methanol and 0.01% butylated hydroxytoluene (Sigma) as an antioxidant. Lipids were extracted by the method of Folch. The fractions of total phospholipids (PH), triacylglycerols (TAG), diacylglycerols (DAG) and free fatty acids (FFA) were separated by thin-layer chromatography (TLC) according to Roemen and van der Vusse (29). Lipid class standards were spotted on the outside lanes of the TLC plate to enable localization of the sample lipid classes. The gel bands corresponding to the standards were scrapped off the plates, transferred into fresh tubes and then transmethylated in 14% methanolic boron trifluoride (Sigma) at 100°C for either 2 (FFA), 10 (DAG) or 30 minutes (PH and TAG). The content of resulting fatty acid methyl esters was determined by means of gas-liquid chromatography as previously described in detail (25). Plasma FFA composition was determined using the same method as for myocardial FFA. Plasma TAG concentration was measured with the Serum Triglyceride Determination Kit (Sigma).
Glycogen content
The content of glycogen in the heart was determined following digestion of the samples in 30% KOH at 100°C for 20 min. Glycogen was then precipitated with ethanol from an aliquot of the digest and assayed by the anthrone method of Carroll
et al. (6).
2-deoxyglucose uptake by the heart
The uptake of 2-deoxyglucose was determined as described by Turinsky
et al.
(31), with the exception that [3H]mannitol instead of [
14C]sucrose
was used to measure the extracellular space. The rats were anesthetized and
1 mCi of 2-deoxy-D-[1-
14C]glucose (specific activity
56 mCi/mmol, NEN Life Science Products) and 5 mCi of [1-
3H]mannitol
(specific activity 26.3 mCi/mmol, NEN Life Science Products) per 100g body weight
were administered into the tail vein. Samples of the cardiac muscle and the
blood were taken 25 min later. The samples were then digested in 1M NaOH followed
by neutralization with 1M HCl. The scintillation cocktail (Ultima Gold, Packard)
was added and the radioactivity was counted. The 2-deoxyglucose uptake was calculated
as the difference between the total myocardial radioactivity and the radioactivity
of the myocardial extracellular space.
Estimation of palmitate oxidation rate in the heart
The rats were anesthetized and 5mCi of [
14C]palmitate
(specific activity 55 mCi/mmol, PerkinElmer) bound to albumin per 100g body
weight was injected into the tail vein. Samples of the heart’s left ventricle
and blood were taken 10 minutes after administration of the radiolabeled palmitate.
The rate of myocardial palmitate oxidation was estimated from the radioactivity
of the acid-soluble metabolites of [
14C]palmitate
(acetyl-CoA, acetylcarnitine and citric acid cycle intermediates) determined
as described by Demaison
et al. (10). The index of cardiac palmitate
oxidation (
Rpox) was calculated according
to Oakes
et al. (27):
Rpox = C
FA
x m
B / C
B
where C
FA is the plasma FFA concentration, mB
is the tissue content of acid-soluble products of [
14C]palmitate
oxidation and C
B is the plasma concentration
of free [
14C]palmitate. Total plasma FFA concentration
was determined using the Wako NEFA C kit (Wako Chemicals). In order to measure
the content of plasma free [
14C]palmitate plasma
lipids were extracted and separated by means of TLC as described above. Radioactivity
of the gel band corresponding to the FFA standard was then counted.
Myocardial expression of PPAR
and
The analysis of the content of PPAR

,
and

protein was carried out with the use of Western blot technique. Briefly, protein
extracts (25 µg) were fractionated on a 10% polyacrylamide gel, transferred
to nitrocellulose membranes, and probed with the antibodies specific to each
PPAR isofom (Santa Cruz Biotechnology). After incubation with the secondary
alkaline phosphatase-conjugated antibody (Sigma) protein bands were scanned
and quantified using a Gel Doc EQ system (Bio-Rad).
Statistical analysis
All data are presented as means ± SD. Statistical comparisons were made by using two-way ANOVA followed by Newman-Keuls post-hoc test. If variances were heterogeneous among groups (Levene’s test, P<0.05), Welch ANOVA and Dunnett’s T3 post-hoc test were used instead. P<0.05 was considered statistically significant. One, two, or three symbols indicate a significant difference at the P<0.05, P<0.01, or P<0.001 levels, respectively.
RESULTS
General features of the experimental animals
High-fat feeding resulted in higher weight gain compared to the standard chow
fed group, however, the difference did not reach statistical significance (
Table
2). Administration of WY-14643 did not affect weight gain in either diet
group. High-fat diet elevated plasma FFA and decreased plasma TAG concentration
(
Table 2). Administration of PPAR

agonist to the animals fed on the standard chow reduced plasma FFA and TAG concentration
by 27 and 15%, respectively. On the contrary, in the high-fat fed rats plasma
FFA and TAG concentration was increased after WY-14643 treatment by 23 and 45%,
respectively.
Table 2. Weight gain and plasma lipids in the experimental groups. |
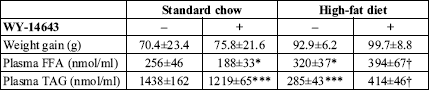 |
Values are means ± SD
(n=10). FFA – free fatty acids, TAG – triacylglycerols. * significant
difference vs. the untreated group fed on the standard chow, †
significant difference vs. the untreated group fed on the high-fat diet. |
Content of myocardial lipids
Administration of WY-14643 induced opposite effects on the total intramyocardial
FFA content in the rats fed standard (43% reduction) and high-fat diet (46%
increase) (
Fig. 1). These changes were, however, limited to SAT and MUFA
as the level of PUFA in this fraction was not affected by PPAR

agonist in either group. High-fat di
et alone decreased the total content
of myocardial FFA by 29% which was a result of reduced level of SAT and MUFA
in this fraction. However, the change reached statistical significance only
in the case of MUFA.
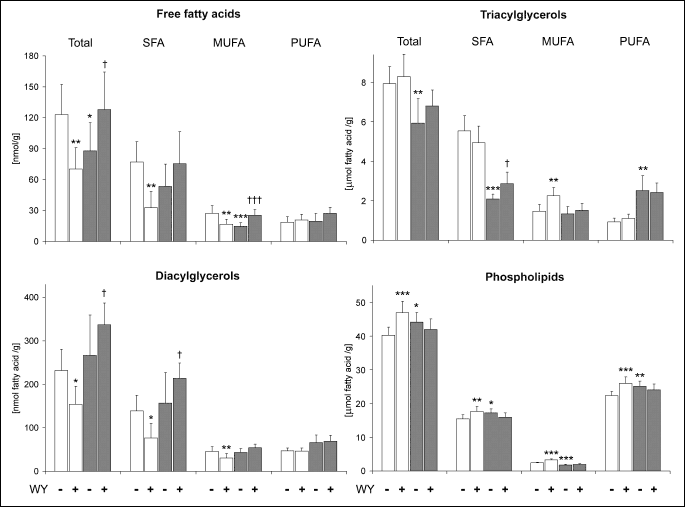 |
Fig.
1. Effects of WY-14643 (WY) and high-fat diet on the myocardial content of fatty acids in the fractions of diacylglycerols, triacylglycerols, phospholipids and free fatty acids. Rats were fed either a standard chow (white bars) or a high-fat diet (grey bars) for three weeks and were treated or not with WY-14643 in a dose of 3 mg/kg/d. Values are means ± SD, n=10. * significant difference vs. the untreated group fed on the standard chow, † significant difference vs. the untreated group fed on the high-fat diet, SFA – saturated fatty acids, MUFA – monounsaturated fatty acids, PUFA – polyunsaturated fatty acids. |
WY-14643-induced effects on the total DAG-fatty acid content in the heart also
depended on dietary fat intake (
Fig. 1). In the group fed standard chow
PPAR

agonist decreased
DAG level by 34%, whereas in the high-fat fed rats the compound induced a 26%
increase in the myocardial DAG content. These changes were a result of alterations
in the level of saturated and monounsaturated DAG species. On the other hand,
high-fat di
et alone affected neither total DAG-fatty acid content nor
the level of individual groups of DAG-fatty acids.
PPAR

agonist did
not produce statistically significant changes in the total content of TAG-fatty
acids in either group (
Fig. 1). However, in the high-fat fed rats WY-14643
induced a trend towards increased TAG level resulting from elevated content
of saturated TAG species. High-fat di
et alone markedly decreased the
level of saturated TAG-fatty acids (by 62%), whereas the content of PUFA in
this fraction was simultaneously elevated 2.7-fold. The net effect of these
changes was a 25% reduction in the total TAG content.
Administration of WY-14643 to the animals fed standard chow increased the content
of all groups of PH-fatty acids which resulted in a 17% elevation in the total
level of PH-fatty acids in the heart (
Fig. 1). However, in the high-fat
fed group the compound had no effect on either total PH-fatty acid content or
the level of individual groups of PH-fatty acids. High-fat di
et alone
increased the total content of PH-fatty acids by 10% which was a consequence
of elevation in the level of SAT and PUFA in this fraction. It should be noted,
however, that the content of PH-MUFA was simultaneously decreased by 26%.
Fatty acid composition of myocardial PH and plasma FFA
WY-14643 exerted similar effects on myocardial PH fatty acid composition in
both diet groups (
Table 3). In general, these changes were reflected
by increased percentage of MUFA (with the exception of palmitoleic acid) and
elevated n6/n3-PUFA ratio. The latter effect was a result of simultaneous decrease
in the percentage of n3-PUFA (with the exception of

-linolenic
acid) and increase in the total percentage of n6-PUFA. However, it should be
noted that in the group fed standard chow the increase in the total percentage
of n6-PUFA was a consequence of elevated percentage of arachidonic acid, whereas
in the high-fat fed rats it resulted from increased percentage of linoleic acid.
Table 3. Effect of WY-14643 on the fatty acid composition of myocardial phospholipids. |
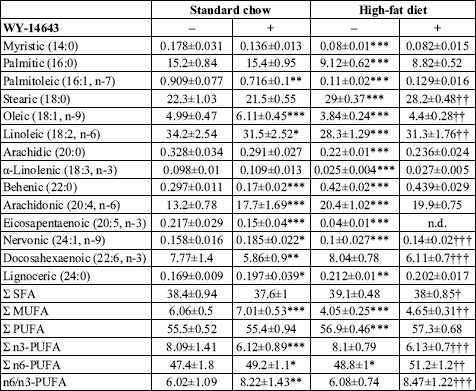 |
Values are mol % ± SD
(n=10). SFA – saturated fatty acids, MUFA – monounsaturated fatty acids,
PUFA – polyunsaturated fatty acids, n.d. – not detected. * significant
difference vs. the untreated group fed on the standard chow, † significant
difference vs. the untreated group fed on the high-fat diet. |
High-fat di
et alone induced profound changes in the fatty acid composition
of myocardial PH. Among all investigated fatty acids only the percentage of
docosahexaenoic acid remained stable. In general, high-fat feeding decreased
the percentage of all MUFA and slightly elevated the total percentage of n6-PUFA.
In the latter group of PH-fatty acids linoleic acid was to a large extent replaced
by arachidonic acid. Among n3-PUFA the percentage of

-linolenic
and eicosapentaenoic acid was markedly decreased by high-fat diet whereas the
percentage of docosahexaenoic acid remained stable.
In general, WY-14643 exerted moderate effect on plasma FFA. It should be noted,
however, that the changes observed in the standard chow fed rats were more pronounced
(
Table 4). The common effect of PPAR

agonist in both diet groups was the increase in the percentage of oleic acid.
The compound did not induce statistically significant changes in the percentage
of any group of free fatty acids in the high-fat fed animals. However, in the
standard chow fed rats a profound reduction in the total percentage of n3-PUFA
resulting from decreased percentage of a-linolenic and docosahexaenoic acid
was observed. There was also a strong trend towards increased n6/n3-PUFA ratio
in this group which, however, did not reach statistical significance (P=0,28).
Table 4. Effect of WY-14643 on the fatty acid composition of plasma free fatty acids. |
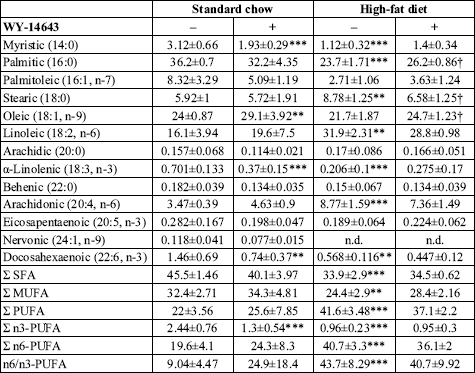 |
Values are mol % ± SD
(n=10). SFA – saturated fatty acids, MUFA – monounsaturated fatty acids,
PUFA – polyunsaturated fatty acids, n.d. – not detected. * significant
difference vs. the untreated group fed on the standard chow, † significant
difference vs. the untreated group fed on the high-fat diet. |
High-fat di
et alone induced marked changes in the composition of plasma FFA. In general, saturated, monounsaturated and n-3 polyunsaturated fatty acids were to a large extent replaced by n6-PUFA, the percentage of which has doubled. In consequence the n6/n3-PUFA ratio increased over 4-fold.
2-deoxyglucose uptake, glycogen content and palmitate oxidation in the heart
WY-14643 did not affect myocardial 2-deoxyglucose uptake in either group, whereas,
high-fat died alone decreased it by 68% (
Fig. 2a). Administration of
PPAR

agonist elevated
glycogen content in the heart by 78 and 43% in rats fed on the standard and
the high-fat diet, respectively (
Fig. 2b). In the standard chow fed rats
WY-14643 decreased the index of cardiac palmitate oxidation (
Rpox)
by 37%, whereas in the high-fat fed animals it was not affected by the drug
(
Fig. 2c).
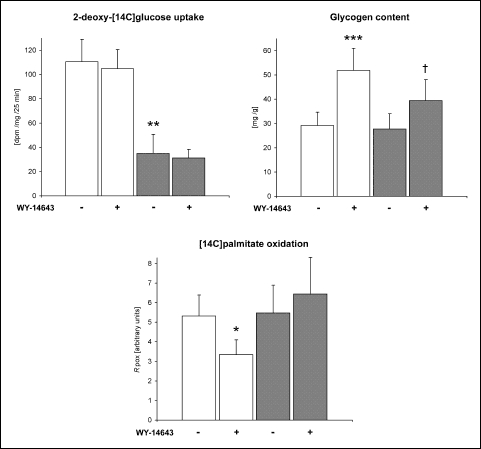 |
Fig. 2. Effects of WY-14643
and high-fat diet on basal 2-deoxy-[14C]glucose
uptake by the heart, myocardial glycogen content and [14C]palmitate
oxidation rate in the heart. Rats were fed either a standard chow (white
bars) or a high-fat diet (grey bars) for three weeks and were treated
or not with WY-14643 in a dose of 3 mg/kg/d. Values are means ± SD, n=6.
* significant difference vs. the untreated group fed on the standard chow,
† significant difference vs. the untreated group fed on the high-fat diet,
Rpox – index of cardiac [14C]palmitate
oxidation. |
Myocardial expression of PPAR
and d
High-fat di
et alone moderately increased the myocardial content of PPAR

and

protein (
Fig. 3). WY-14643 administration did not affect the content
of PPAR

protein
in the heart of the standard chow fed animals whereas in the high-fat fed rats
the drug induced a modest decrease in the receptor protein expression. On the
other hand, the myocardial level of PPAR

was decreased by WY-14643 treatment in both diet groups (by 17 and 29% in rats
fed on the standard and high-fat diet, respectively).
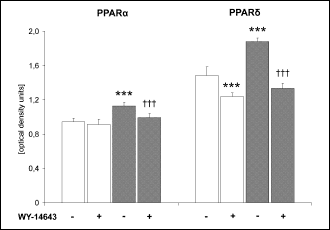 |
Fig. 3. Effects of WY-14643
and high-fat diet on the level of PPAR
and PPAR
protein in the heart. Rats were fed either a standard chow (white bars)
or a high-fat diet (grey bars) for three weeks and were treated or not
with WY-14643 in a dose of 3 mg/kg/d. Values are means ± SD, n=6. * significant
difference vs. the untreated group fed on the standard chow, †
significant difference vs. the untreated group fed on the high-fat diet. |
DISCUSSION
To the best of our knowledge this is the first report describing effects of PPAR

activator on the content and fatty acid composition of all major glycerolipid classes in the heart. We showed that effect of WY-14643 on the level of myocardial lipids to a large extent depended on dietary fat intake. Administration of the drug to the rats fed on the standard chow markedly reduced FFA and DAG content in the heart, whereas in the high-fat fed animals the opposite effect was observed. Similar findings were reported in our previous paper where WY-14643 was shown to differently affect sphingolipid metabolism in the heart of rats fed standard chow and high-fat diet (3). In general, the above mentioned changes in the myocardial content of FFA and DAG reflected effects of the drug on plasma lipid concentration. Namely, in the rats fed on the standard chow PPAR

agonist exerted hypolipidemic effect, whereas in the high-fat fed animals plasma lipid concentration was increased. This suggests that WY-14643-induced changes in the myocardial content of FFA and DAG were indirect and secondary to alterations in plasma lipid availability. This hypothesis is also supported by the effect of the PPAR

agonist on cardiac palmitate oxidation. In the standard chow fed animals WY-14643 paradoxically decreased the content of acid soluble metabolites of radiolabeled palmitate in the heart which was likely a consequence of the lipid lowering action of the drug. Therefore, increased fatty acid flux through the oxidative pathway could not contribute to the observed decrease in myocardial FFA and DAG. On the other hand, in the high-fat fed rats WY-14643 did not affect palmitate oxidation in the heart which further indicates that the increase in the content of myocardial lipids in this group was a consequence of elevated availability of blood-borne fatty acids. Unfortunately, there is no literature data on effects of PPAR

agonists on the myocardial content of FFA and DAG. In our experiment PPAR

activation did not affect TAG level in the heart of rats fed on either diet which is in line with other studies (2, 24, 32).
It was shown that high-fat feeding can reduce spontaneous physical activity in rats (26). Since we do not have data on the level of physical activity in different experimental groups, we cannot exclude the possibility that this factor might have had an impact on parameters of lipid metabolism measured in our study. It is therefore plausible that diet-dependent differences in the effect of WY-14643 on myocardial lipid metabolism were a consequence of PPAR

activation on the background of not only distinct fat intake, but also distinct level of physical activity.
As already mentioned in the introduction numerous experiments conducted on cultured cardiac myocytes revealed that PPAR

activation induces expression of a wide array of genes involved in fatty acid catabolic pathways. However, most
in vivo studies have shown little or no effect of PPAR

agonists on myocardial expression of genes regulated by this receptor (1, 9, 20). Moreover, synthetic PPAR

activators were paradoxically found to decrease cardiac fatty acid oxidation rate in murine models of diabetes and diet-induced obesity (1, 2) which is in line with the results of our study. This discrepancy between
in vitro and
in vivo studies is generally thought to be a consequence of the fact that
in vivo PPAR

transcriptional activity is limited by receptor expression rather than ligand availability. Indeed, in the study by Finck
et al. (13) it was shown that WY-14643 upregulates PPAR

-dependent genes in the heart of mice with cardiac-restricted overexpression of PPAR

but not in their wild-type littermates. Taking together, it is very likely that effects of WY-14643 on the content of myocardial lipids observed in our study were indirect and secondary to changes in systemic lipid metabolism.
An interesting finding of our study is that WY-14643 administration induced
a decrease in the myocardial expression of PPAR

in both diet groups. This effect could rather not be secondary to changes in
systemic lipid metabolism since PPAR

agonist induced opposite effects on plasma lipid concentration in each diet
group. Experiments on cultured rodent cardiomyocytes revealed that PPAR

and PPAR

regulate similar set of genes involved in fatty acid catabolism (34). It was
also shown that cardiomyocyte-restricted PPAR

knockout mice exhibit markedly lower myocardial fatty acid oxidation rate (7).
It is therefore tempting to speculate that in the heart selective PPAR

stimulation may negatively affect PPAR

expression and in consequence also its transcriptional activity. This mechanism
might contribute to the lack of increase in the myocardial palmitate oxidation
rate following WY-14643 administration.
In the present experiment PPAR

activator did not affect basal myocardial glucose uptake which is in line with the study by Finck
et al. (13) showing no effect of WY-14643 on GLUT1 expression in the murine heart. Moreover, Ye
et al. (35) reported that WY-14643 did not modify myocardial glucose uptake during euglycemic-hyperinsulinemic calmp in high-fat fed rats. A new finding of our study is that PPAR

agonist markedly increased glycogen content in the heart. Mandard
et al. (19) reported that murine glycogen synthase 2 (Gys-2) gene contains active PPAR response elements and that PPAR

is a transcriptional regulator of Gys-2 expression in liver and white adipose tissue. They also showed that WY-14643 markedly increases Gys-2 expression in isolated rat hepatocytes. Therefore, it is plausible that PPAR

agonist-induced elevation in the myocardial glycogen content observed in our study was a consequence of its increased synthesis resulting from higher glycogen synthase expression. However, it is not known whether the muscle isoform of this enzyme is also under transcriptional control of PPAR

.
WY-14643 exerted profound influence on the fatty acid composition of myocardial phospholipids. These changes were to a large extent independent from dietary fat intake and in general, included increased percentage of MUFA and replacement of n3-PUFA by n6-PUFA. Our results are in contrast to an earlier study by Tian
et al. (30) that found markedly decreased n6/n3-PUFA ratio in myocardial lipids in rats treated with clofibrate. It should be noted, however, that they determined fatty acid composition of total lipid extracts, whereas in our study it was analyzed in the fraction of phospholipids isolated by means of TLC. This likely contributed to the observed discrepancy.
WY-14643-induced elevation in the percentage of MUFA in the myocardial phospholipids
was most likely a consequence of increased availability of oleic acid, since
administration of the drug increased the percentage of this fatty acid in plasma
FFA in both diet groups. Increased percentage of oleic acid in plasma lipids
after treatment with a PPAR

agonist was observed also by Christiansen
et al. (8). This action of
PPAR

activators
is thought to be a result of increased expression of liver stearoyl-CoA desaturase
(23). Although WY-14643 induced similar changes in phospholipid PUFA composition
in both diet groups the mechanism of its action seems to be different in each
group. In the standard chow fed rats alterations in the myocardial phospholipid
PUFA composition generally reflected those observed in plasma FFA which indicates
that the effect of PPAR

agonist was indirect and secondary to changes in systemic PUFA metabolism. Indeed
it was shown that PPAR

activation stimulates
5-
and
6-desaturase
expression as well as n6-PUFA synthesis in the rat liver (16, 21). On the other
hand, in the high-fat fed animals WY-14643 did not affect either n6/n3-PUFA
ratio or the percentage of any of plasma free PUFA which suggests direct action
of the drug on PUFA metabolism in the heart.
There is abundant evidence that n3-PUFA are beneficial to the cardiovascular system. Clinical trials showed that intake of fish oil rich in eicosapentaenoic and docosahexaenoic acid reduces the mortality due to myocardial infarction. Moreover, experimental studies on rodents revealed that enrichment of myocardial membranes with n3-PUFA has anti-arrhythmic effect, reduces ischemic damage of the heart and increases post-ischemic recovery (22). Therefore, decreased contribution of n3-PUFA in the myocardial phospholipids that was observed in our study after PPAR

agonist administration should be considered as an unfavourable effect of the drug that might have detrimental effects on the heart. Our results indicate that the aforementioned effect of WY-14643 is not transient, since it was still present after 14 days of drug administration. However, additional experiments using more chronic treatment are necessary to fully address this issue.
In summary, we found that WY-14643 induced reciprocal effects on the myocardial content of FFA and DAG in rats fed on the standard and high-fat diet. These changes followed alterations in plasma lipid concentration which along with the lack of increase in palmitate oxidation rate in the heart implies indirect action of PPAR

agonist on cardiac lipid metabolism. Administration of WY-14643 had profound influence on fatty acid composition of myocardial phospholipids with the most notable effect being increased n6/n3-PUFA ratio. This action of PPAR

activator might be detrimental to the heart since n3-PUFA possess cardioprotective and antiarrhythmic properties.
Acknowledgements:
This work was supported by the Medical University of Bialystok grants no. 3-18828
and 4-18620.
Conflict of interests: None declared.
REFERENCES
- Aasum E, Cooper M, Severson DL, Larsen TS. Effect of BM 17.0744, a PPAR alpha; ligand, on the metabolism of perfused hearts from control and diabetic mice. Can J Physiol Pharmacol 2005; 83: 183-190.
- Aasum E, Khalid AM, Gudbrandsen OA, How OJ, Berge RK, Larsen TS. Fenofibrate modulates cardiac and hepatic metabolism and increases ischemic tolerance in diet-induced obese mice. J Mol Cell Cardiol 2008; 44: 201-209.
- Baranowski M, Blachnio A, Zabielski P, Gorski J. PPAR alpha agonist induces the accumulation of ceramide in the heart of rats fed high-fat diet. J Physiol Pharmacol 2007; 58: 57-72.
- Barger PM, Kelly DP. PPAR signaling in the control of cardiac energy metabolism. Trends Cardiovasc Med 2000; 10: 238-245.
- Campbell FM, Kozak R, Wagner A et al. A role for peroxisome proliferator-activated receptor alpha (PPAR alpha) in the control of cardiac malonyl-CoA levels: reduced fatty acid oxidation rates and increased glucose oxidation rates in the hearts of mice lacking PPAR alpha are associated with higher concentrations of malonyl-CoA and reduced expression of malonyl-CoA decarboxylase. J Biol Chem 2002; 277: 4098-4103.
- Carroll NV, Longley RW, Roe JH. The determination of glycogen in liver and muscle by use of anthrone reagent. J Biol Chem 1956; 220: 583-593.
- Cheng L, Ding G, Qin Q et al. Cardiomyocyte-restricted peroxisome proliferator-activated receptor-delta deletion perturbs myocardial fatty acid oxidation and leads to cardiomyopathy. Nat Med 2004; 10:1245-1250.
- Christiansen RZ, Norseth J, Christiansen N. The effect of clofibrate on heart and plasma lipids in rats fed a diet containing rapeseed oil. Lipids 1979; 14: 614-618.
- Cook WS, Yeldandi AV, Rao MS, Hashimoto T, Reddy JK. Less extrahepatic induction of fatty acid beta-oxidation enzymes by PPAR alpha. Biochem Biophys Res Commun 2000; 278: 250-257.
- Demaison L, Blet J, Sergiel JP, Gregoire S, Argaud D. Effect of dietary polyunsaturated fatty acids on contractile function of hearts isolated from sedentary and trained rats. Reprod Nutr Dev 2000; 40: 113-125.
- Djouadi F, Weinheimer CJ, Kelly DP. The role of PPARalpha as a “lipostat”
transcription factor. Adv Exp Med Biol 1999; 466: 211-220.
- Escher P, Braissant O, Basu-Modak S, Michalik L, Wahli W, Desvergne B. Rat PPARs: quantitative analysis in adult rat tissues and regulation in fasting and refeeding. Endocrinology 2001; 142: 4195-4202.
- Finck BN, Lehman JJ, Leone TC et al. The cardiac phenotype induced by PPAR alpha overexpression mimics that caused by diabetes mellitus. J Clin Invest 2002; 109: 121-130.
- Forman BM, Chen J, Evans RM. Hypolipidemic drugs, polyunsaturated fatty acids, and eicosanoids are ligands for peroxisome proliferator-activated receptors alpha and delta. Proc Natl Acad Sci USA 1997; 94: 4312-4317.
- Hopkins TA, Sugden MC, Holness MJ, Kozak R, Dyck JR, Lopaschuk GD. Control of cardiac pyruvate dehydrogenase activity in peroxisome proliferator-activated receptor-alpha transgenic mice. Am J Physiol Heart Circ Physiol 2003; 285: H270-H276.
- Kawashima Y, Musoh K, Kozuka H. Peroxisome proliferators enhance linoleic acid metabolism in rat liver. Increased biosynthesis of omega 6 polyunsaturated fatty acids. J Biol Chem 1990; 265: 9170-9175.
- Kiec-Wilk B, Dembinska-Kiec A, Olszanecka A, Bodzioch M, Kawecka-Jaszcz K. The selected pathophysiological aspects of PPARs activation. J Physiol Pharmacol 2005; 56: 149-162.
- Leone TC, Weinheimer CJ, Kelly DP. A critical role for the peroxisome proliferator-activated receptor alpha (PPAR alpha) in the cellular fasting response: the PPAR alpha-null mouse as a model of fatty acid oxidation disorders. Proc Natl Acad Sci USA 1999; 96: 7473-7478.
- Mandard S, Stienstra R, Escher P et al. Glycogen synthase 2 is a novel target gene of peroxisome proliferator-activated receptors. Cell Mol Life Sci 2007; 64: 1145-1157.
- Martin G, Schoonjans K, Lefebvre AM, Staels B, Auwerx J. Coordinate regulation of the expression of the fatty acid transport protein and acyl-CoA synthetase genes by PPAR alpha and PPAR gamma activators. J Biol Chem 1997; 272: 28210-28217.
- Matsuzaka T, Shimano H, Yahagi N et al. Dual regulation of mouse Delta(5)- and Delta(6)-desaturase gene expression by SREBP-1 and PPAR alpha. J Lipid Res 2002; 43: 107-114.
- McLennan PL, Owen AJ, Slee EL, Theiss ML. Myocardial function, ischaemia and n-3 polyunsaturated fatty acids: a membrane basis. J Cardiovasc Med (Hagerstown) 2007; 8 (Suppl 1): S15-S18.
- Miller CW, Ntambi JM. Peroxisome proliferators induce mouse liver stearoyl-CoA desaturase 1 gene expression. Proc Natl Acad Sci USA 1996; 93: 9443-9448.
- Morgan EE, Rennison JH, Young ME et al. Effects of chronic activation of peroxisome proliferator-activated receptor alpha or high-fat feeding in a rat infarct model of heart failure. Am J Physiol Heart Circ Physiol 2006; 290: H1899-H1904.
- Nawrocki A, Gorski J. Effect of plasma free fatty acid concentration on the content and composition of the free fatty acid fraction in rat skeletal muscles. Horm Metab Res 2004; 36: 601-606.
- Novak CM, Kotz CM, Levine JA. Central orexin sensitivity, physical activity, and obesity in diet-induced obese and diet-resistant rats. Am J Physiol Endocrinol Metab 2006; 290: E396-E403.
- Oakes ND, Thalen P, Aasum E et al. Cardiac metabolism in mice: tracer method developments and in vivo application revealing profound metabolic inflexibility in diabetes. Am J Physiol Endocrinol Metab 2006; 290: E870-E881.
- Pascoe WS, Storlien LH. Inducement by fat feeding of basal hyperglycemia in rats with abnormal beta-cell function. Model for study of etiology and pathogenesis of NIDDM. Diabetes 1990; 39: 226-233.
- Roemen TH, van der Vusse GJ. Application of silica gel column chromatography in the assessment of non-esterified fatty acids and phosphoglycerides in myocardial tissue. J Chromatogr 1985; 344: 304-308.
- Tian Q, Grzemski FA, Panagiotopoulos S, Ahokas JT. Peroxisome proliferator-activated receptor alpha agonist, clofibrate, has profound influence on myocardial fatty acid composition. Chem Biol Interact 2006; 160: 241-251.
- Turinsky J, Bayly BP, O’Sullivan DM. 1,2-Diacylglycerol and ceramide levels in rat skeletal muscle and liver in vivo. Studies with insulin, exercise, muscle denervation, and vasopressin. J Biol Chem 1990; 265: 7933-7938.
- Vikramadithyan RK, Hirata K, Yagyu H et al. Peroxisome proliferator-activated receptor agonists modulate heart function in transgenic mice with lipotoxic cardiomyopathy. J Pharmacol Exp Ther 2005; 313: 586-593.
- Watanabe K, Fujii H, Takahashi T et al. Constitutive regulation of cardiac fatty acid metabolism through peroxisome proliferator-activated receptor alpha associated with age-dependent cardiac toxicity. J Biol Chem 2000; 275: 22293-22299.
- Yang Q, Li Y. Roles of PPARs on regulating myocardial energy and lipid homeostasis. J Mol Med 2007; 85: 697-706.
- Ye JM, Doyle PJ, Iglesias MA, Watson DG, Cooney GJ, Kraegen EW. Peroxisome proliferator-activated receptor (PPAR)-alpha activation lowers muscle lipids and improves insulin sensitivity in high fat-fed rats: comparison with PPAR-gamma activation. Diabetes 2001; 50: 411-417.