STEREOSELECTIVITY OF TRADINTEROL'S INHIBITION ON PROLIFERATION
OF AIRWAY SMOOTH MUSCLE CELLS INDUCED BY ACETYLCHOLINE
THROUGH SUPPRESSING Ca2+ SIGNALING
2School of Pharmaceutical Engineering, Shenyang Pharmaceutical University, Shenyang, China;
3Key Laboratory of Structure Based Drug Design and Discovery, Ministry of Education, Shenyang, China
INTRODUCTION
Nowadays, asthma is one of the most common chronic diseases characterized by airway inflammation, airway hyperresponsiveness and airway remodeling. Airway smooth muscle, once believed to be just a contractile tissue, has many other biological and functional properties including airway remodeling and amplification of airway inflammation in addition to its contraction (1). Histopathological examination of bronchi in asthmatic patients found serious inflammatory cell infiltration and airway wall thickening compared with that in normal people (2). Although many types of phenotypic changes of airway cells are involved in airway remodeling, airway smooth muscle cells (ASMCs) proliferation is the most important factor that leads to airway narrowing (3-5). Therefore, ASMCs may be a new potential target for asthma therapy (6, 7).
ASMCs proliferation is mediated by various factors, one of which, intracellular Ca2+ signaling, may play a key role in regulation of multiple cellular functions, such as contraction, relaxation, secretion of inflammatory mediators, migration and proliferation (8). It is demonstrated that intracellular Ca2+ accumulation is mainly dependent on the release from intracellular stores including sarcoplasmic reticulum (SR) and mitochondria and Ca2+ influx across the plasma membrane (9, 10). Furthermore, inositol 1,4,5-trisphosphate receptor (IP3) is produced when agonists, like acetylcholine (ACh), bind to G-protein coupled receptors (GPCRs). It combines and activates IP3R on SR membrane, causing release of Ca2+ from SR. Depletion of Ca2+ in SR activates SOC via the SR Ca2+-sensing protein stromal-interacting molecule 1 (STIM1), as it associates with the plasmalemmal protein Orai 1, which causes the latter to form channels mediating SOCE (11-14). Numerous studies confirm that STIM1/Orai1-mediated SOCE signal pathway is connected with ASMCs proliferation (15-17).
Glucocorticoids, β2 adrenergic agonist and theophylline are still major bronchodilators for the treatment of asthma so far. Previous study demonstrates that glucocorticoids show inhibitory effect on cultured human ASMCs proliferation induced by mitogen. Dixon et al. also found that dexamethasone (25 – 250 nM) significantly suppressed the proliferation in vitro through inhibiting DNA synthesis induced by beta-hexosaminidase A (Hex A, 50 nM) in bovine ASMCs (18).
However, the effect of the anti-asthma drugs especially β2 agonists on airway remodeling is rarely studied. Gao et al. (19) found that the long-acting β2 agonist salmeterol but not salbutamol inhibited ASMCs proliferation by suppressing the opening of SOC promoted by IL-13. The study conducted by Kassel et al. also proved that the selective β2-agonists albuterol and salmeterol inhibited the ASMCs proliferation stimulated by epidermal growth factors via a PKA-independent mechanism (20). Moreover, it is considered that the mechanisms of action between glucocorticoids and β2 agonists in inhibiting Ca2+ signaling and proliferation are different from each other (18, 20, 21). Fogli et al. found that β2-agonists had a synergistic interaction with PPARγ agonists on ASMCs proliferation and this combined treatment promoted cell cycle arrest at the G1/S transition phase (22).
SPFF, dl-2-(3-chloro-4-amino-5-trifluoromethyl) phenyl-2- tertbutylamine-ethanol dihydrochloride, an innovative drug of Shenyang Pharmaceutical University, has excellent relaxation on ASM and anti-asthmatic effect (23-25). Results from a multicenter, randomized, double-blind, dose-finding clinical research showed that it could significantly improve the lung function of patients with bronchial asthma, and was well-tolerated (26). Evidence from preclinical in vivo study demonstrated the different activity of (–)SPFF, (+)SPFF and (±)SPFF in improving the pulmonary function in the animals with asthma (23). Previous study of (±)SPFF revealed that the mechanism of its suppression of intracellular Ca2+ in ASMCs might be involved in IP3R pathway (27). Latest research has uncovered that ACh is not only a contraction agonist by increasing intracellular Ca2+ (28), but also plays an important role in inflammation and remodeling (29). In this study, ACh was used as a stimulator to induce ASMCs proliferation and intracellular Ca2+ increasing. The effect of SPFF isomers and the mechanisms of their action were investigated.
MATERIAL AND METHODS
Animals
Male or female Hartley guinea pigs weighing 150 – 200 g were provided by the Experimental Animal Center of Shenyang Pharmaceutical University. Animals were bred in a facility controlled by temperature (26 ± 3°C), relative humidity (50 ± 5%) and light (14 hours of light and 10 hours of dark), with free access to food and water added with vitamin C. All experimental procedures in this study were carried out in accordance with the internationally accepted principles and the Guidelines for the Care and Use of Laboratory Animals of Shenyang Pharmaceutical University, Shenyang, China.
Drugs and chemicals
(±)SPFF, (–)SPFF and (+)SPFF were supplied by the Pharmaceutical Engineering Department, Shenyang Pharmaceutical University (Shenyang, Liaoning, China, enantiomeric excess, > 99%). ACh was purchased from Sinopharm Chemical Reagent Co., Ltd (Shanghai, China). Dulbecco's modified Eagle's medium (DMEM) and Hanks' balanced salt solution (HBSS) were purchased from Gibco-BRL (Carlsbad, CA, USA). Type I collagenase was from Beijing Solarbio Science & Technology Co., Ltd. (Beijing, China). Fetal bovine serum (FBS) was produced by Tianjin Hualida Biotechnology Co., Ltd. (Tianjin, China). Triton X-100 and MTT were obtained from Ameresco (Solon, OH, USA). Mouse anti-α-smooth muscle actin (α-SMA), 5% bovine serum albumin (BSA), streptavidin-biotin complex (SABC) immunohistochemical staining kit, and 3,3 N-diaminobenzidine tetrahydrochloride (DAB) chromogenic reagent kit were all purchased from Wuhan Boster Biological Technology Co., Ltd. (Wuhan, China). Fura-2/AM, Fluo-3/AM as well as FITC-labeled goat anti-mouse IgG were from Beyotime Institute of Biotechnology (Haimen, Jiangsu, China). Propidium iodide (PI) kit and RNase A were purchased from Wanlei Biological Technology Co., Ltd. (Shenyang, China).
Cell cultures
Freshly dispersed guinea pig ASMCs were prepared following the steps described (30). Briefly, guinea pigs were anesthetized, and tracheal smooth muscle was removed and minced, and then dispersed in Ca2+-free HBSS under 4°C. After removing the connective tissue and epithelium, the tracheal smooth muscle strips were cut into small pieces and were resuspended in 2% type I collagenase for 20 min (37°C, 5% CO2). The pieces were washed with Ca2+-free HBSS and then moved into a culture flask (Corning Life Sciences, Tewksbury MA, USA) with DMEM containing 20% fetal bovine serum (FBS, Biological Industries, BI), 100 units/ml penicillin G, 100 µg/ml streptomycin (Gibco, USA) and 2 mM L-glutamine. The media was changed every 72 hours. After confluence, the guinea pig ASMCs were digested with 0.25% trypsin - 0.02% ethylene diamine tetraacetic acid (EDTA) solution in 0.01 M PBS and then suspended in 15% FBS-DMEM. To exclude the contamination of epithelial cells and fibroblasts, the cells' homogeneity was confirmed with smooth muscle-specific α-actin by immunocytochemistry and immunofluorescence. It was found that over 95% the cell preparation was composed of ASMCs (30). The cells of Generation 3 to 6 were used in the experiments.
Human ASMCs, purchased from Abace Biological Technology Co. Ltd. (Beijing, China), were maintained as recommended by the manufacturer in DMEM/HIGH GLUCOSE (HyClone, USA) supplemented with 10% FBS, 100 units/ml of penicillin G and 100 µg/ml streptomycin. The cells of Generation 3 to 8 were used in the experiments.
Cell proliferation assay
Evaluation of guinea pig ASMCs proliferation with MTT assay
Cells seeded in 96-well plates at density of 3 × 104 per well were treated with ACh (100 µM) after cultured in 15% FBS-containing DMEM for 12 hours. And then the cells were incubated with different concentration of test drugs including (±)SPFF (2 or 40 µM), (–)SPFF (2 or 40 µM), (+)SPFF (2 or 40 µM), or 2-APB (90 µM) and NiCl2 (100 µM) as well, respectively, for 24 or 48 hours under the condition of 37°C with 5% CO2. The proliferation of guinea pig ASMCs was determined with MTT assay. The amount of MTT formazan was quantified by measuring optical density (OD) at 492 nm with the Varioskan Flash. The percentage of increase in OD was calculated according to the following equation: the percentage of increase in OD = (ODSPFF – ODcontrol)/ODSPFF × 100%.
Evaluation of human ASMCs proliferation with flow cytometry analysis
Synchronized human ASMCs were treated with different concentration of test drugs including (±)SPFF (2,40 and 80 µM), (–)SPFF (2,40 and 80 µM) and (+)SPFF (2,40 and 80 µM), the pharmacological tools such as 2-APB (90 µM) and NiCl2 (100 µM) as well as the combination of (±)SPFF (40 µM) + 2-APB (90 µM), (–)SPFF (40 µM) + 2-APB (90 µM) and (+)SPFF (40 µM) + 2-APB (90 µM), respectively, for 72 hours after being incubated with ACh (100 µM) for 30 min. The cells were harvested after being rinsed with PBS for several times and thereafter resuspended in the solution of PI Kit according to the manufacture's protocol. They were incubated for 30 min at 4°C in dark. The sample was analyzed with the flow cytometry (FACSCalibur, BD, SanJose, California, 95131-1807, USA).
Intracellular Ca2+ measurement
The measurement was carried out according to the procedures described in the previous study (30). Briefly, cells were incubated with 5 µM Fluo-3/AM for 45 min at 37°C to be loaded with the indicator when the intracellular Ca2+ was determined with the flow cytometry, alternatively the cells were incubated with 5 µM Fura-2/AM when the intracellular Ca2+ was detected with Varioskan Flash (Thermo Scientific, Rockford, IL, USA) or Ca2+ fluorescent images were taken in ultraviolet region under an inverted microscope (IX71, Olympus, Tokyo, Japan). Cells were washed with Ca2+-free HBSS after being loaded with Fluo-3/AM or Fura-2/AM to determine Ca2+ fluorescent intensity.
The Fura-2/AM-loaded cells were moved into black 96-well culture plates (Corning, Tewksbury MA 01876, USA) at a density of about 2 × 104 cells per well for the determination of Ca2+ fluorescent intensity (F) with the Varioskan Flash under the condition of 340 nm excitation wavelength and 510 nm emission wavelength. The percentage of increase in fluorescence was calculated according to the equation: The percentage of increase in fluorescence = (F340 SPFF – F340 control)/F340 SPFF × 100%. The rate of calcium inhibition was calculated according to the equation: calcium inhibitory rate (%) = (F340 ACh – F340 SPFF)/F340 ACh × 100%. Moreover, Ca2+ fluorescent images were obtained from the Fluo-2/AM-loaded cells in ultraviolet region under the inverted microscope.
In addition, intracellular Ca2+ in the Fluo-3/AM-loaded cells were determined at 37°C with the flow cytometry under the condition of 488 nm excitation wavelength and 525 nm emission wavelength, from which GM can be obtained. The percentage of increase in GM was measured according to the equation: the percentage of increase in GM = (GM340 SPFF – GM340 control)/GM340 SPFF × 100%.
SPFF treatment
Guinea pig ASMCs were prepared and seeded in a 1.5 ml microcentrifuge tube (Gene Era Biotech, USA) at a density of
1 × 106 cells/ml. 10–3~10–7 mM (±)SPFF, (–)SPFF or (+)SPFF was respectively applied on the cells for 60 min. And then, 100 µM of ACh was added to induce intracellular Ca2+ for 5 min. The intracellular Ca2+ was determined with the Varioskan Flash, inverted microscope and flow cytometry, respectively.
Application of the pharmacology tools
IP3R blocker/SOC blocker
Guinea pig ASMCs were collected as described above and then incubated with (–)SPFF (0.03 µM), (+)SPFF (0.2 µM), (±)SPFF (0.06 µM) alone, or plus NiCl2 (SOC blocker, 100 µM)/2-APB (IP3R blocker, 90 µM) for 60 min (Table 1). Then, ACh (100 µM) was added and the incubation system was mixed for approximately 5 min. After 30 min, the 2nd ACh was added to testify whether the response of ACh was kept at the same level or not due to receptor desensitization (Table 1).
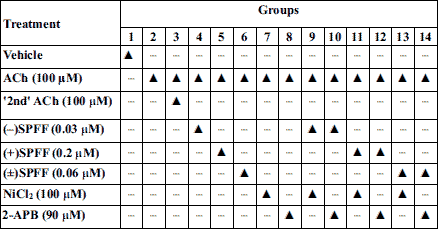
Ca2+-free treatment and application of extracellular calcium
Similar steps as described above were followed except that the experiment was conducted in Ca2+-free environment when the inhibitory effect of the test drugs on SOC-mediated intracellular Ca2+ was under investigation. Briefly, ASMCs were incubated with (–)SPFF (0.03 µM), (+)SPFF (0.2 µM), (±)SPFF (0.06 µM), NiCl2 (100 µM), and 2-APB (90 µM) for 60 min, respectively. CaCl2 (10 mM) was added as extracellular calcium in order to induce the release of calcium in SR (31). Intracellular Ca2+ was determined with the Varioskan Flash.
Statistical analysis
All data are expressed as mean ± S.E.M. and statistical comparisons among groups were performed with one-way analysis of variance (ANOVA) followed LSD. *P < 0.05 is considered to be significant and P > 0.05 is non-significant throughout this paper. The figures were plotted with the software GraphPad Prism 5 (San Diego, CA, USA).
RESULTS
(–)SPFF/(±) SPFF but (+)SPFF inhibits acetylcholine-induced proliferation of guinea pig ASMCs
As shown in Fig. 1, 100 µM ACh promotes guinea pig ASMCs proliferation and the percentage increase in OD is time-dependent, it is from 34.39 ± 2.32 at T24 h shown in Fig. 1A (compared with 15% FBS control, #P > 0.05) to 45.87 ± 2.81% at T48 h in Fig. 1B (*P < 0.05). (–)SPFF (40 µM) and (±)SPFF (40 µM) reduced the percentage increase in OD to 32.19 ± 7.52% (*P < 0.05) and 32.02 ± 5.22% (*P < 0.05), respectively (Fig. 1B). 90 µM 2-APB, an IP3R blocker, suppressed the proliferation significantly (Fig. 1A and Fig. 1B). The suppression was not significantly different between (–)SPFF (40 µM) and 2-APB (90 µM) (#P > 0.05) or (±)SPFF (40 µM) and 2-APB (90 µM) (#P > 0.05) at T48 h (Fig. 1B). (+)SPFF (40 µM) or NiCl2 (100 µM) merely reduced the percentage increase in OD to 40.97 ± 6.68% (P = 0.39) or 35.96 ± 5.65% (P = 0.096) (Fig. 1B).
![]() |
Fig. 1. Effect of (–)SPFF, (±)SPFF and (+)SPFF on ACh-promoted guinea pig ASMCs proliferation. The cells were treated with different drugs for 24 h (Fig. 1A) or 48 h (Fig. 1B) respectively. The absorbance at 492 nm obtained from MTT assay with the Varioskan Flash and the percentage increase in OD was calculated following the description in Section 2.4. Data were expressed as mean ± S.E.M. based on at least three independent experiments. * P < 0.05 compared to the group treated with ACh and # P > 0.05 compared between the groups indicated in this figure, by one-way ANOVA followed LSD with SPSS 16.0. |
(–)SPFF/(±) SPFF but (+)SPFF inhibits acetylcholine-induced proliferation of human ASMCs
Cell cycle analysis with flow cytometry was applied to evaluate the anti-proliferative effects of (–)SPFF, (±)SPFF and (+)SPFF on human ASMCs. As shown in Fig. 2, the rate of cells in Phase S over all the cells treated with ACh was significant higher than that of control (*P < 0.05). This effect of ACh was significantly suppressed by (–)SPFF, (±)SPFF but (+)SPFF. The combining effect of (–)SPFF or (±)SPFF with 2-APB is significant (*P < 0.05) as well. There was no significant difference (#P > 0.05) between the effects of (–)SPFF/(±)SPFF alone and the combining effect of (–)SPFF or (±)SPFF with 2-APB. The three drugs had no significant influence on the rate of cells in Phase G1 and G2 (Table 2).
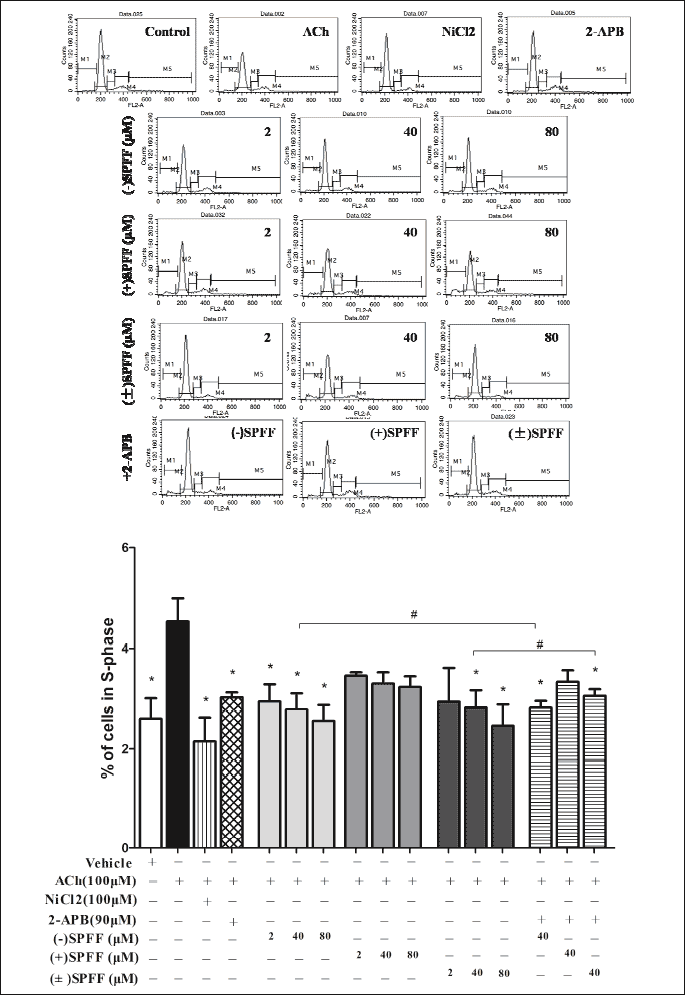
# P > 0.05 compared between the groups indicated in the figure. The upper figure showed data that were obtained with the flow cytometry (M2 = G1; M3 = S; M4 = G2). Data in the column figure were calculated from the flow cytometry data, which represented the percentage of cells in Phase S over the total cells.
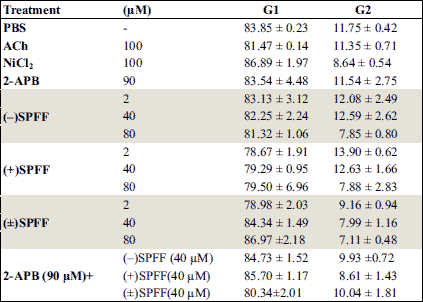
Suppression of the elevation of intracellular Ca2+ induced by acetylcholine
As shown in Fig. 3, ACh (100 µM) induced a significant increment of Ca2+ fluorescent intensity (*P < 0.05, compared with the vehicle control). Pre-incubation with (–)SPFF, (+)SPFF and (±)SPFF suppressed the increment of Ca2+ fluorescent intensity in a dose-dependent manner. As far as (–)SPFF and (±)SPFF were concerned (Fig. 3A and Fig. 3C), the percentage increase in fluorescence reduced significantly at the concentration of 10–3, 10–4 and 10–5 mM when compared with that treated with ACh alone. IC50 of the three compounds scaled from low to high were (–)SPFF, (±)SPFF and (+)SPFF (Table 3).
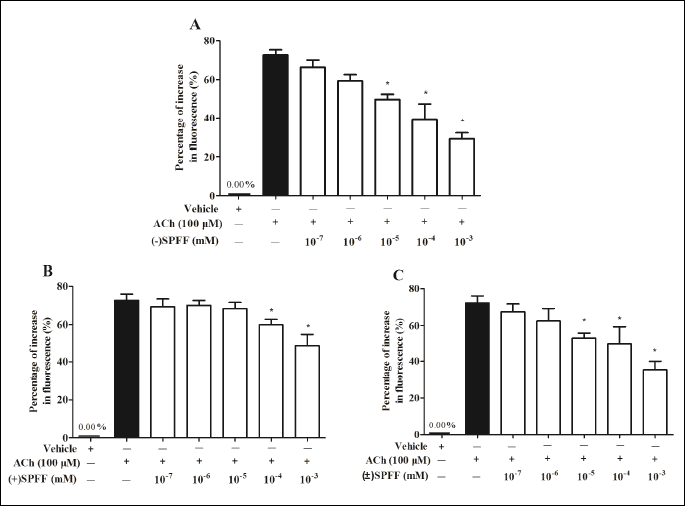

The results from the inverted microscope (Fig. 4) as well as the flow cytometry (Fig. 5) are consistent with those from the varioskan flash. In Fig. 4, more fluorescent spots and higher fluorescent intensity can be seen in the sample treated with ACh alone than that of the vehicle control. (–)SPFF, (+)SPFF and (±)SPFF show dose-dependent reduction of ACh-induced fluorescent intensity at the concentration of 10–3, 10–5, 10–7 mM, respectively. In Fig. 5, pretreatment with (–)SPFF, (+)SPFF and (±)SPFF suppressed dose-dependently the geometric mean value of range M1 in diagram of flow cytometry, which represents Ca2+ fluorescent intensity. (–)SPFF and (±)SPFF at the concentration of both 10–3 and 10–5 mM showed significant suppression of the percentage increase in GM induced by ACh, but only at 10–3 mM a significant effect can be observed with regard to (+)SPFF. These dose-dependent inhibitory effects of the drugs were shown intuitively in the flow cytometry figures at the upper part of Fig. 5.
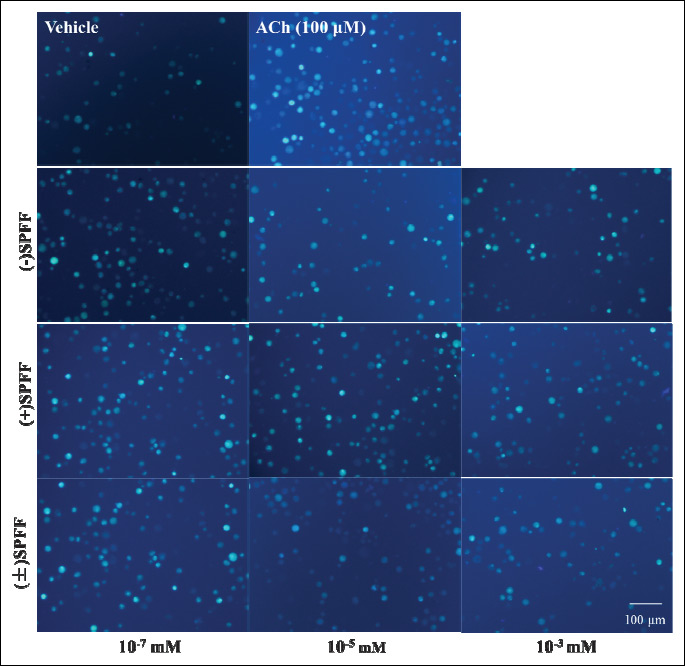
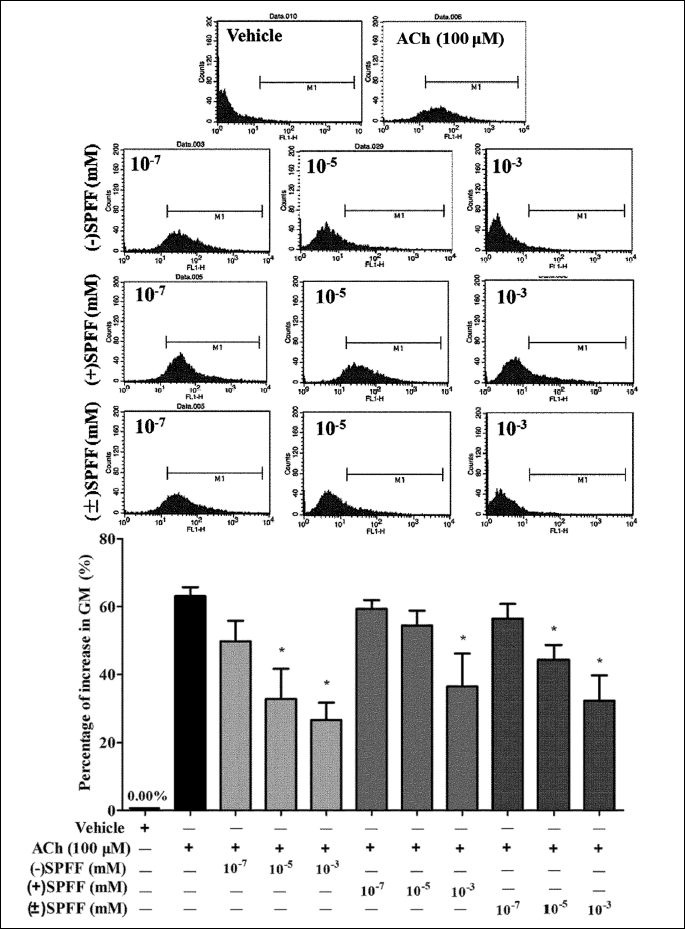
(–)SPFF/(±) SPFF but (+)SPFF inhibits Ca2+ influx via IP3R-mediated SOCE
As shown in Fig. 6, 100 µM of ACh induced Ca2+ fluorescent increase, and repeated administration led to similar peak response (#P > 0.05). NiCl2 (SOC blocker, 100 µM) or 2-APB (IP3R blocker, 90 µM) alone showed significant inhibitory effect (*P < 0.05), while (–)SPFF, (+)SPFF and (±)SPFF alone significantly suppressed the Ca2+ fluorescent increase induced by ACh (*P < 0.05). When (–)SPFF and (±)SPFF, respectively, were simultaneously given with 2-APB or NiCl2, no additive effect was observed. There were no significant difference between (–)SPFF/(±)SPFF alone group and the simultaneous combination group with NiCl2/2-APB (#P > 0.05). This finding implies that (–)SPFF/(±) SPFF and 2-APB might act through a common pathway. However, no similar occurrence was found in terms of (+)SPFF, and there was significant difference (*P < 0.05) between (+)SPFF alone and its combined group with NiCl2 or 2-APB.
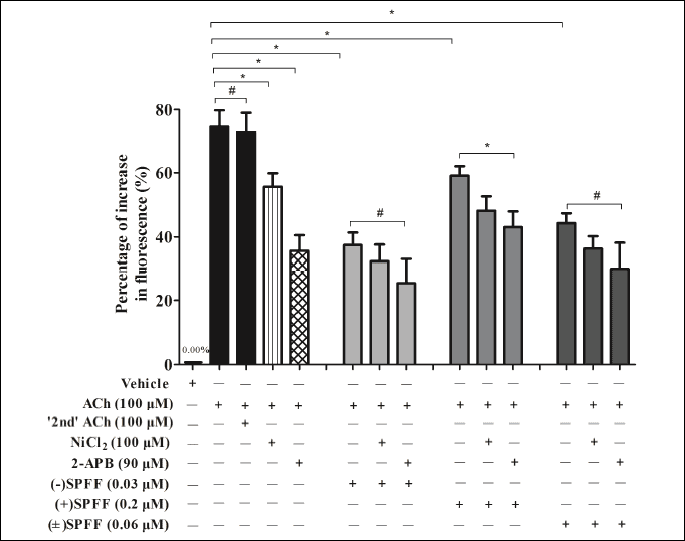
To confirm the above mentioned association, further study was carried out under the condition of Ca2+-free treatment and then extracellular calcium (10 mM CaCl2) was added. Results in Fig. 7 showed that NiCl2, the SOC blocker, could directly inhibit Ca2+ fluorescent increase induced by CaCl2, i.e. the influx of extracellular Ca2+ via SOC. Moreover, the high concentration of 2-APB inhibits the influx probably through interfering SOC. This IP3R blocker can make Ca2+ increase in SR because of its suppression of Ca2+ release via IP3R from SR, which then triggers SOC-mediated extracellular Ca2+ influx through STIM1 signaling. Contrary to (+)SPFF, (–)SPFF and (±)SPFF can also significantly inhibit the percentage of fluorescence increase in induced by CaCl2 (Fig. 7). Considering the results observed above, it is possible that (–)SPFF and (±)SPFF inhibit the ACh-induced Ca2+ elevation in ASMCs through suppressing IP3R to interfere the influx of extracellular Ca2+ mediated by SOC.
![]() |
Fig. 7. (–)SPFF/(+)SPFF/(±)SPFF suppression of Ca2+ increase in fluorescence under the condition of Ca2+-free treatment and then application of extracellular calcium (10 mM CaCl2). NiCl2, a SOC blocker, could directly inhibit the Ca2+ fluorescent increase induced by CaCl2. High concentration of 2-APB also inhibited the Ca2+ influx. Intracellular calcium was loaded with Fura-2/AM and Ca2+ fluorescent intensity was determined with the varioskan flash under the condition of 340 nm excitation wavelength and 510 nm emission wavelength. Increase in fluorescence (%) was expressed as mean ± S.E.M. obtained from three independent experiments. *P < 0.05 compared to the group treated with 10 mM CaCl2 by one-way ANOVA followed LSD with SPSS 16.0. |
![]() |
Fig. 8. Chemical structures of classical β2 adrenoceptors, epinephrine and isoprenoline, and SPFF isomers. |
DISCUSSION
It has been demonstrated that SPFF, a new type of β2 agonist, significantly reduces airway resistance. The in vivo study reveals the significant increased effect of SPFF on pulmonary overflow and lung compliance when compared to that of the traditional β2 agonist (25). Moreover, SPFF also exhibits significant suppression of intracellular Ca2+ in ASMCs reported in the previous work (27). ASM relaxation induced by the activation of β2 adrenergic is reported to be accompanied by the decrease of Ca2+ oscillations and Ca2+ sensitivity. ASM relaxation elicited by nitric oxide is also relevant to the suppression of Ca2+ release through IP3R and the decreased frequency of agonist-induced Ca2+ oscillations (32). It was documented that nitric oxide (NO) in respiratory tract contributed to local host defence, modulated ciliary motility and served as an aerocrine mediator beside its responsibility for the ASM relaxation (33). Moreover, substance P (SP), a peptide, induces bronchoconstriction in subjects with asthma through stimulating neurokinin-1 (NK-1) receptor, the pathway of which is involved in the promotion of calcium mobilization (34). It is also reported that the interaction between the peptide and the receptor leads to cell proliferation and SP antagonist has the potential to be developed as anti-proliferation agent (35).
It is known that ASMCs proliferation contributes to the structural changes of respiratory tract. Ca2+ signaling is one of the important factors to remodel respiratory tract through mediating the migration and ASMCs proliferation (8). It has been recently uncovered that the activation of SOC-mediated Ca2+ signaling pathway is an important factor in airway remodeling (15, 16). It is reported that ACh makes Ca2+ in SR deplete when it stimulates the elevation of intracellular Ca2+ release through IP3R. This IP3R-mediated Ca2+ depletion induces the activation of SOC channels on plasma membrane (36, 37). Pharmacological blockers of SOC channels, including 2-APB and gadolinium, are often used in the investigation (38, 39). Gao et al. proves that IL-13 induces ASMCs proliferation by increasing Ca2+ release and SOC entry as well (19). Glucocorticoids and β2 agonists have different characteristics from each other in regard to their suppressive effects on Ca2+ signaling and proliferation stimulated by the interleukin (19). Dekkers et al. found that glucocorticosteroids synergized with β2 agonists synergized to inhibit airway remodeling induced with platelet-derived growth factor (PDGF) (10 ng/ml), collagen type I (50 µg/ml), and insulin (1 µM), respectively (40).
PDGF has been proved to promote ASMCs proliferation and migration by mediating STIM1 and Orai1. It is indicated that STIM1 and Orai1 might be a potential target for improving ASMCs proliferation which often occurred concomitantly in chronic asthma (16). The rearrangement phosphatidylinositol-4,5-bisphosphate at endoplasmic reticulum-plasma membrane junctions during the formation of STIM1-Orai1 clusters was testified to facilitate STIM1 targeting to these junctions and promote the stable recruitment of Orai1 (41). Amiodarone was previously found to lead to the increase of [Ca2+]i (42). And it induced the phospholipidosis in rat alveolar epithelial cells after medium-term oral administration (43). These effects may contribute to the drug's severe pulmonary toxicity although it needs to be illustrated whether the increase of [Ca2+]i elicited by the drug is related to the accumulation of phosphatidylinositol-4,5-bisphosphate and STIM1/Orai1-mediated SOCE.
Data in the current study showed that 100 µM of ACh increased the proliferation of guinea pig ASMCs significantly after T48 h incubation with the cells. The result is in consistent with that in recent publication, in which ACh is demonstrated to contribute to airway remodeling (29). (–)SPFF/(±)SPFF but (+)SPFF suppressed the proliferation promoted by ACh significantly. Moreover, human ASMCs were used in the present study to investigate the potential therapeutic effect of the SPFF stereoisomers. The time of cell exposure to the drugs was also extended to 72 hours in this study to further assess the inhibitory ability of the drugs on human ASMCs proliferation, to which flow cytometry analysis was applied. It was showed that the SPFF stereoisomers could inhibit the percentage of the cells in Phase S over all the cells stimulated with ACh and had no influence on that in Phase G1 and Phase G2. The stereoselective activities of SPFF on the proliferation of human ASMCs evaluated by the flow cytometry analysis are consistent with the result from guinea pig ASMCs.
It is mentioned that both ACh and SOCE participated in regulating ASMCs proliferation (19, 29). In this study, (–)SPFF/(±)SPFF was found to inhibit ASMCs proliferation induced with ACh obviously and there was no significantly different effect between (–)SPFF/(±)SPFF and 2-APB, an IP3R blocker, at high concentration. Considering that 2-APB is well known to intervene in the influx of extracellular Ca2+ mediated through SOC by means of inhibiting the IP3R significantly (44, 45), whether SOC-mediated Ca2+ signaling is involved in the proliferation inhibited by the two compounds was investigated. 2-APB (90 µM) and NiCl2 (100 µM) were used to examine if there was a similarity in the modes of action among (–)SPFF, (±)SPFF, 2-APB (90 µM) and NiCl2 (100 µM). In addition, it is discovered that (–)SPFF, (+)SPFF and (±)SPFF took different inhibitory effects on the ACh-induced intracellular Ca2+ elevation. The emergence of different inhibitory effects may be caused by the different stereoselective activities of SPFF isomers. Further study illustrated that no significant difference in fluorescence inhibition between (–)SPFF/(±) SPFF alone and the combined application of (–)SPFF/(±) SPFF with 2-APB. This result is in consistent with the modes of action in the drugs' inhibiting ASMCs proliferation. (–)SPFF and (±)SPFF also showed their inhibitory effect on Ca2+ influx induced with 10 mM Ca2+, which was further evidence that (–)SPFF, (±)SPFF and 2-APB (90 µM) might act through a common pathway in the suppression of intracellular Ca2+ elevation induced with ACh. Therefore, it is inferred that (–)SPFF and (±)SPFF, similar to 2-APB, probably interferes SOC-mediated Ca2+ influx by inhibiting IP3R on SR.
In what mode that a ligand such as a drug binds to its specific receptor can be confirmed by chemically detecting co-crystal structure of ligand and receptor, which can reveal the interaction between drug and receptor and is crucial for achieving the drug's high potency (46). Unfortunately, study relevant to the co-complex of SPFF with active domain of β2 receptor has not been conducted yet. As a result, it is hard to judge whether the findings in the present study that the different stereoselective activities lead to different suppression levels of ACh-induced intracellular Ca2+ signaling and the proliferation are specific or can be extended to the whole class of β2 agonist. It is mentioned that SPFF is not exactly the same with classical β2 agonist. The drug is regarded as a novel and atypical one not only because its chemical structure differs greatly from those of the classical β2 agonists (Fig. 8) but also the pharmacological properties are quite unlike (23, 25). Moreover, to understand the difference of the SPFF isomers in potency, it is thought that a high activity is generally derived from a closely matched binding and a great force between the configuration of a drug and its corresponding receptor. The affinity of (–)SPFF to β2 receptor in radioligand binding assay was previously found 6 and 164 fold greater than that of (±)SPFF and (+)SPFF (25). The different stereoselectivities of the isomers found in the present study are mostly due to the drugs' stereostructures (Fig. 8).
Clinical trial showed SPFF's beneficial therapy for patients with persistent mild to moderate asthma and had good effects on patients with obvious symptoms of airway obstruction. Its action differs completely from the same with that of traditional β2 receptor agonists. This study found that SPFF significantly reduced [Ca2+]i in ASMCs and proliferation induced with ACh. The mechanism of the action was probably involved in the regulation of IP3R on SR. In addition, it was found that the role of SPFF in inhibiting the transient elevation of [Ca2+]i might be related to SR Ca2+ ATPase (SERCA) (data not shown here). Based on the results and the mechanisms of airway remodeling, it is deduced that this drug is clinically helpful to prevent the patients with chronic asthma from airway remodeling.
In conclusion, (–)SPFF and (±)SPFF contribute to the suppression of ASMCs proliferation through IP3R/SOC-mediated Ca2+ signaling. Different stereoselective activities of (–)SPFF, (+)SPFF and (±)SPFF may lead to the different levels of the inhibition of ACh-induced intracellular Ca2+ signaling and the proliferation, which suggests that these therapies may have distinct effects on airway remodeling in asthma.
Abbreviations: ACh, acetylcholine; ASMCs, airway smooth muscle cells; BSA, bovine serum albumin; DMEM, Dulbecco's modified eagle's medium; FBS, fetal bovine serum; GM, geo mean; GPCRs, G-protein coupled receptors; HBSS, Hanks' balanced salt solution; IP3R, inositol 1,4,5-trisphosphate receptor; α-SMA, mouse anti-α-smooth muscle actin; DAB, 3,3 N-diaminobenzidine tetrahydrochloride; OD, optical density; PDGF, platelet-derived growth factor, PI, propidium iodide; SR, sarcoplasmic reticulum; SOC, store-operated channel; SOCE, store-operated Ca2+ entry; SABC, streptavidin-biotin complex; STIM1, stromal-interacting molecule 1.
Acknowledgements: This study was supported by grants from the Education Department of Liaoning (No. L2015531) and training program of innovation for undergraduates in Shenyang Pharmaceutical University.
Xirui Song and Hongyun Wang contributed equally to this work.
Conflict of interests: None declared.
REFERENCES
- Prakash YS. Airway smooth muscle in airway reactivity and remodeling: what have we learned? Am J Physiol Lung Cell Mol Physiol 2013; 305: L912-L933.
- Eskandari M, Kuschner WG, Kuhl E. Patient-specific airway wall remodeling in chronic lung disease. Ann Biomed Eng 2015; 43: 2538-2551.
- Girodet PO, Ozier A, Bara I, Tunon de Lara JM, Marthan R, Berger P. Airway remodeling in asthma: new mechanisms and potential for pharmacological intervention. Pharmacol Ther 2011; 130: 325-337.
- Allen JC, Seidel P, Schlosser T, Ramsay EE, Ge Q, Ammit AJ. Cyclin D1 in ASM cells from asthmatics is insensitive to corticosteroid inhibition. J Allergy (Cairo) 2012; 2012: 307838. doi: 10.1155/2012/307838.
- Kaminska M, Foley S, Maghni K, et al. Airway remodeling in subjects with severe asthma with or without chronic persistent airflow obstruction. J Allergy Clin Immunol 2009; 124: 45-51.
- Dowell ML, Lavoie TL, Solway J, Krishnan R. Airway smooth muscle: a potential target for asthma therapy. Curr Opin Pulm Med 2014; 20: 66-72.
- Lazaar AL. Airway smooth muscle: new targets for asthma pharmacotherapy. Expert Opin Ther Targets 2002; 6: 447-459.
- Mahn K, Ojo OO, Chadwick G, Aaronson PI, Ward JP, Lee TH. Ca2+ homeostasis and structural and functional remodelling of airway smooth muscle in asthma. Thorax 2010; 65: 547-552.
- Jude JA, Wylam ME, Walseth TF, Kannan MS. Calcium signaling in airway smooth muscle. Proc Am Thorac Soc 2008; 5: 15-22.
- Koopmans T, Anaparti V, Castro-Piedras I, et al. Ca2+ handling and sensitivity in airway smooth muscle: emerging concepts for mechanistic understanding and therapeutic targeting. Pulm Pharmacol Ther 2014; 29: 108-120.
- Liou J, Kim ML, Heo WD, et al. STIM is a Ca2+ sensor essential for Ca2+-store-depletion-triggered Ca2+ influx. Curr Biol 2005; 15: 1235-1241.
- Roos J, DiGregorio PJ, Yeromin AV, et al. STIM1, an essential and conserved component of store-operated Ca(2+) channel function. J Cell Biol 2005; 169: 435-445.
- Feske S, Gwack Y, Prakriya M, et al. A mutation in Orai1 causes immune deficiency by abrogating CRAC channel function. Nature 2006; 441: 179-185.
- Peel SE, Liu B, Hall IP. ORAI and store-operated calcium influx in human airway smooth muscle cells. Am J Respir Cell Mol Biol 2008; 38: 744-749.
- Zou JJ, Gao YD, Geng S, Yang J. Role of STIM1/Orai1-mediated store-operated Ca2+ entry in airway smooth muscle cell proliferation. J Appl Physiol 1985 2011; 110: 1256-1263.
- Spinelli AM, Gonzalez-Cobos JC, Zhang X, et al. Airway smooth muscle STIM1 and Orai1 are upregulated in asthmatic mice and mediate PDGF-activated SOCE, CRAC currents, proliferation, and migration. Pflugers Arch 2012; 464: 481-492.
- Suganuma N, Ito S, Aso H, et al. STIM1 regulates platelet-derived growth factor-induced migration and Ca2+ influx in human airway smooth muscle cells. PLoS One 2012; 7: e45056. doi: 10.1371/journal.pone.0045056.
- Dixon ER, Weinberg JA, Lew DB. Effect of dexamethasone on bovine airway smooth muscle cell proliferation. J Asthma 1999; 36: 519-525.
- Gao YD, Zou JJ, Zheng JW, et al. Promoting effects of IL-13 on Ca2+ release and store-operated Ca2+ entry in airway smooth muscle cells. Pulm Pharmacol Ther 2010; 23: 182-189.
- Kassel KM, Wyatt TA, Panettieri RA, Toews ML. Inhibition of human airway smooth muscle cell proliferation by beta 2-adrenergic receptors and cAMP is PKA independent: evidence for EPAC involvement. Am J Physiol Lung Cell Mol Physiol 2008; 294: L131-L138.
- Stewart AG, Fernandes D, Tomlinson PR. The effect of glucocorticoids on proliferation of human cultured airway smooth muscle. Br J Pharmacol 1995; 116: 3219-3226.
- Fogli S, Stefanelli F, Picchianti L, et al. Synergistic interaction between PPAR ligands and salbutamol on human bronchial smooth muscle cell proliferation. Br J Pharmacol 2013; 168: 266-275.
- Pan H, Li Q, Pan L, et al. Stereoselective activity of 2-(4-amino-3-chloro-5- trifluomethyl-phenyl)-2-tert-butylamino-ethanol hydrochloride to improve the pulmonary function in asthma. Biomed Rep 2014; 2: 539-544.
- Gan LL, Wang MW, Cheng MS, Pan L. Trachea relaxing effects and beta2-selectivity of SPFF, a newly developed bronchodilating agent, in guinea pigs and rabbits. Biol Pharm Bull 2003; 26: 323-328.
- Hao Z, Zhang Y, Pan L, et al. Comparison of enantiomers of SPFF, a novel beta2-adrenoceptor agonist, in bronchodilating effect in guinea pigs. Biol Pharm Bull 2008; 31: 866-872.
- Kong L, Yuan Y, Shao Y, Sun S, Liu J, Kang J. Efficacy and tolerability of tratinterol hydrochloride tablets in bronchial asthma: a multicenter, randomized, double-blind, dose-finding clinical trial. Clin Ther 2014; 36: 1195-1204.
- Liu J, Zhang Y, Li Q, et al. An improved method for guinea pig airway smooth muscle cell culture and the effect of SPFF on intracellular calcium. Mol Med Rep 2014; 10: 1309-1314.
- Ressmeyer AR, Bai Y, Delmotte P, et al. Human airway contraction and formoterol-induced relaxation is determined by Ca2+ oscillations and Ca2+ sensitivity. Am J Respir Cell Mol Biol 2010; 43: 179-191.
- Kistemaker LE, Gosens R. Acetylcholine beyond bronchoconstriction: roles in inflammation and remodeling. Trends Pharmacol Sci 2015; 36: 164-171.
- Song XR, Zhao C, Dai CL, et al. Suppression of the increasing level of acetylcholine-stimulated intracellular Ca2+ in guinea pig airway smooth muscle cells by mabuterol. Biomed Rep 2015; 3: 778-786.
- Ghayur MN, Gilani AH, Janssen LJ. Ginger attenuates acetylcholine-induced contraction and Ca2+ signalling in murine airway smooth muscle cells. Can J Physiol Pharmacol 2008; 86: 264-271.
- Perez-Zoghbi JF, Bai Y, Sanderson MJ. Nitric oxide induces airway smooth muscle cell relaxation by decreasing the frequency of agonist-induced Ca2+ oscillations. J Gen Physiol 2010; 135: 247-259.
- Bencova A, Vidan J, Rozborilova E, Kocan I. The impact of hypertonic saline inhalation on mucociliary clearance and nasal nitric oxide. J Physiol Pharmacol 2012; 63: 309-313.
- Maghni K, Michoud MC, Alles M, et al. Airway smooth muscle cells express functional neurokinin-1 receptors and the nerve-derived preprotachykinin-A gene. Am J Respir Cell Mol Biol 2003; 28: 103-110.
- Munoz M, Recio S, Rosso M, Redondo M, Covenas R. The antiproliferative action of [D-Arg(1), D-Phe(5), D-Trp(7,9), LEU(11)] substance P analogue antagonist against small-cell- and non-small-cell lung cancer cells could be due to the pharmacological profile of its tachykinin receptor antagonist. J Physiol Pharmacol 2015; 66: 421-426.
- Putney JW. A model for receptor-regulated calcium entry. Cell Calcium 1986; 7: 1-12.
- Putney JW. Capacitative calcium entry revisited. Cell Calcium 1990; 11: 611-624.
- Broad LM, Cannon TR, Taylor CW. A non-capacitative pathway activated by arachidonic acid is the major Ca2+ entry mechanism in rat A7r5 smooth muscle cells stimulated with low concentrations of vasopressin. J Physiol 1999; 517: 121-134.
- Trebak M, Bird GS, McKay RR, Putney JW Jr. Comparison of human TRPC3 channels in receptor-activated and store-operated modes. Differential sensitivity to channel blockers suggests fundamental differences in channel composition. J Biol Chem 2002; 277: 21617-2123.
- Dekkers BG, Pehlic A, Mariani R, Bos IS, Meurs H, Zaagsma J. Glucocorticosteroids and b2-adrenoceptor agonists synergize to inhibit airway smooth muscle remodeling. J Pharmacol Exp Ther 2012; 342: 780-787.
- Sharma S, Quintana A, Findlay GM, et al. An siRNA screen for NFAT activation identifies septins as coordinators of store-operated Ca2+ entry. Nature 2013; 499: 238-242.
- Muend S, Rao R. Fungicidal activity of amiodarone is tightly coupled to calcium influx. FEMS Yeast Res 2008; 8: 425-431.
- Kapatou E, Skyrlas A, Agelaki MG, Pantos C, Kolettis TM, Malamou-Mitsi V. Amiodarone attenuates apoptosis, but induces phospholipidosis in rat alveolar epithelial cells. J Physiol Pharmacol 2010; 61: 671-677.
- Maruyama T, Kanaji T, Nakade S, Kanno T, Mikoshiba K. 2APB, 2-aminoethoxydiphenyl borate, a membrane-penetrable modulator of Ins(1,4,5)P3-induced Ca2+ release. J Biochem 1997; 122: 498-505.
- Bilmen JG, Michelangeli F. Inhibition of the type 1 inositol 1,4,5-trisphosphate receptor by 2-aminoethoxy-diphenylborate. Cell Signal 2002; 14: 955-960.
- Zorn JA, Wang Q, Fujimura E, Barros T, Kuriyan J. Crystal structure of the FLT3 kinase domain bound to the inhibitor quizartinib (AC220). PLoS One 2015; 10: 1-15.
A c c e p t e d : June 14, 2016