Inhalation of oxygen in a higher concentration than present in ambient air is widely used in clinical practice. Long-term oxygen therapy is used in patients with chronic hypoxemia that can occur in several respiratory and cardiac disorders, including chronic obstructive pulmonary diseases (COPD), adult respiratory distress syndrome, and interstitial lung diseases. Oxygen supplementation improves survival, pulmonary hemodynamics, exercise capacity, and improves the quality of live in such patients (1, 2). Unfortunately, with progressing pulmonary dysfunction, increasing concentrations of oxygen are required, which increases the likelihood of additional pulmonary injury due to toxic effects of oxygen (1). The basic mechanism underlying oxygen toxicity appears to be generation of reactive oxygen species (ROS). ROS have the ability to react with and damage essential biomolecules, including enzymes, membrane lipids, and nucleic acids (3).
Toxic influence of high oxygen concentrations on pulmonary function and structures has been known for years (4-6). However, information about the influence of hyperoxia on defensive respiratory reflexes, including the cough reflex, is still limited. On the basis of available information we supposed that hyperoxia alone or in combination with primary lung tissue injury should have a toxic influence on lung tissue, including the airway nerve endings that are responsible for mediation and modulation of cough.
We previously demonstrated a suppressant effect of 100% oxygen breathing for 60 h on chemically-induced cough in awake guinea-pigs (7). The inhibitory effect of pure normobaric oxygen breathing on citric acid-induced cough has been shown in experiments with experimental airway damage induced by either bleomycin or ovalbumin (8, 9). Our previous results suggest that 100% oxygen plays a key role in the development of airway and lung damage, which correlates with a decrease of cough reflex intensity.
There is increasing evidence of protective and preventive effects of antioxidant supplementation in different disorders including chronic respiratory diseases. This raises the possibility that combined dietary antioxidant vitamins could affect oxidant-antioxidant imbalance, diminish oxidative damage, and minimize airway inflammation (10, 11).
There are various options to enhance the lung antioxidant screen. One approach
would simply be to administer antioxidant therapy using vitamin C and vitamin
E, which reduces oxidative stress in patients with COPD (12) and other related
diseases (13). Vitamin E, with the most active compound
alpha-tocopherol,
is a lipophilic chain-breaking antioxidant; it acts by stopping the chain reaction
involved in lipid peroxidation. Vitamin C or L-ascorbic acid is hydrophilic
and may quench radicals in the non-lipid cellular compartments (14). There is
considerable evidence of synergistic interaction between these vitamins. Ascorbic
acid also protects biomembranes against peroxidation by enhancing the activity
of a-tocopherol. Ascorbic acid reduces the tocopheroxyl radical and thereby
restores the radical scavenging activity of
alpha-tocopherol
(15). Thus, it seems a reasonable assumption that concomitant supplementation
with vitamin C and vitamin E might enhance pulmonary antioxidant capacity and
pulmonary defenses against hyperoxia-induced oxygen toxicity.
In this paper we focused on studying the effects of dietary vitamins C and E on airway function in guinea pigs after long-term oxygen therapy. The cough reflex was considered an indicator of airway dysfunction.
MATERIAL AND METHODS
Animals
The study protocol was approved by the Ethics Committee of the Jessenius Faculty of Medicine for animal experiments in Martin and by the State Veterinary and Nutritive Administration of Slovakia according to the veterinary care act.
The study was performed on 24 adult male Trik guinea-pigs weighing 250-350 g.
The animals were purchased from the Department of Experimental Pharmacology
of the Slovak Academy of Science (Dobra Voda, Slovakia). They were kept in the
animal house with food and water
ad libitum and with standard air conditioning
system before starting the experiment. The animals were assigned to one of the
following three protocol groups: antioxidant therapy and hyperoxia group (T-H,
n8) - pretreated with a combination vitamins C and E for 4 wk and subsequently
exposed to 100% O
2 for 60 h, hyperoxic group (H, n=8) - received saline instead
of antioxidants and was exposed to the same 100% O
2
for 60 h, and control group (C, n=8) - exposed to ambient air under the same
condition as the hyperoxic group.
The animals of the T-H group received, daily, with food vitamin C (500 mg/kg; Zentiva, Czech Republic) and vitamin E (300 mg/kg, Biotika, Slovakia) for 4 wk before and two days during hyperoxia. After antioxidant pretreatment, the animals of both T-H group and H group were continuously exposed to 100% oxygen for 60 h. The control group was exposed to ambient air, with otherwise similar protocol.
Exposures either to oxygen or air were performed individually in a sealed glass chamber. Oxygen concentration was periodically monitored by an oxygen analyzer (Permolyt 3, Veb Junkalor, Germany). Other biophysical parameters of the chamber environment were the following: temperature 22-24°C, humidity 55-65%, CO
2 concentration ~0.2 %, and O
2 concentration in ambient air ~21.0%.
Chemically-induced cough
Unanesthetized animals were individually placed into a body plethysmograph box (type 855, Hugo Sachs Elektronic, Germany) and were exposed to citric acid aerosol (Lachema) in doubly increasing concentrations (from 0.05 to 1.6 M) with 30 s inhalation of each concentration of the tussigen. The interval between separate exposures was 1 min. To expose an animal to the aerosol, the head chamber was connected to a jet nebulizer (Pari Provocation Test I, Pari Starneberg, Germany; manufacturer’s specification: output of aerosol - 5 l min-1, particle mass median diameter - 1.2 µm). Cough was distinguished on the basis of plethysmograph airflow changes and measured using a pneumotachograph (Godart, Germany) with a Fleish head (Gould Godart Statham BV, type 18515, No. 1) connected to the head chamber of the plethysmographic box. The airflow was directly registered with the moving pen recorder (Multiscriptor Hellige 21). The appearance of cough also was detected with a microphone placed in the roof of the head chamber and connected to a tape recorder. The airflow signal and cough sound were simultaneously recorded in PC for off-line analysis. The cough response was evaluated on the basis of sudden enhancement of expiratory airflow accompanied by a typical cough sound. The cough sound was analyzed from a self-developed software system according to Xiang et al (16). Differentiation between cough and sneeze was based on spectral analysis of respective sounds (digitalized at a sampling frequency of 11025 Hz). As was shown previously, cough sound differs from sneeze sound by shorter duration, lower frequency of maximal spectral power peak, and a steeper increase of sound intensity at the beginning of sound (16). To quantify the intensity of cough reaction, the cough response was expressed as a total number of coughs during all citric acid challenges.
A control cough challenge was done in all groups of animals (1
st
cough challenge) at the onset of the experiment. The next provocation of cough
was performed after antioxidant treatment before hyperoxia (2
nd
cough challenge), and a final cough challenge was performed at 60 h of exposure
to 100% O
2 or ambient air (3
rd
cough challenge).
Mechanically-induced cough
Cough also was induced by mechanical stimulation of laryngopharyngeal (LPh) and tracheobronchial (TBr) mucosa using a nylon fiber during 5 s in anesthetized animals (urethane, 1.1 g/kg, i.p.) after tracheostomy 1 h after the end of exposure to hyperoxia and chemically-induced cough challenge (17). The number of coughs was then counted from the trace of intrapleural pressure recorded by an electromanometer (Multiscriptor Hellige 21) using intrapleural cannula. Tracheobronchial and laryngopharyngeal cough was analyzed separately. The number of cough efforts (NE) during a cough bout and the intensity of a cough bout (ICB = the sum of all positive deflection of intrapleural pressure during all cough efforts in the cough bout) were used to quantify cough (18).
At the end of the experiment, anesthetized animals were killed by an overdose of anesthesia and samples of tracheal, bronchial and lung tissue were removed, fixed in 10% formalin, dehydrated in sequential alcohol concentrations, and embedded in paraffin. Cross-sectional specimens were made and stained with hematoxylin and eosin. Histopathological assessment was performed by light microscopy. Visual and manual inspection of lung tissue consistency also was performed.
Statistical analysis
The number of coughs is presented as median and interquartile range. Data on the intensity of cough bouts are expressed as means ±SD. The inter-group differences in the cough response at baseline were assessed with one-way analysis of variance (ANOVA). To compare the effects of antioxidant vitamins and hyperoxia two-way ANOVA for repeated measures was employed. The effects of mechanically induced cough were assessed with one-way ANOVA followed by the Duncan multiple range test, if significant differences were detected.
RESULTS
Effects of supplementation with vitamins C and E on citric acid-induced cough in guinea-pigs exposed to 100% O2
We found no significant differences in the citric acid-induced cough among control,
T-H (antioxidant-hyperoxic) group, and H (hyperoxic alone) group of animals
at baseline [5(4.5-6.5)
vs. 5(1.5-7.5)
vs. 7(5.5-8); P=0.27].
Antioxidant supplementation (T-H group) did not significantly alter the number
of chemically-induced coughs compared with H group (hyperoxia only), as studied
before [4(1.5-5.5)
vs. 5(4-8); P=0.72] or after hyperoxic exposure [6(3.5-9.5)
vs. 3(3-4); P=0.77] (
Fig. 1, upper panels). Comparison of the
effects of hyperoxia in both tested groups showed a clear tendency for a decrease
in citric acid-induced cough in the H group and an increase in the T-H group.
When cough responses were analyzed on the individual basis, there was a significant
interaction between antioxidant therapy and hyperoxia (P=0.004) (
Fig. 1,
bottom panel).
 |
Fig. 1.
Effects of hyperoxia on citric acid-induced cough in the group without
antioxidants - hyperoxia only group (H, solid line) and the antioxidant-hyperoxia
group (T-H, broken line). The number of coughs is expressed as median
and interquartile range (upper panels); NS - non-significant. Lower panel
shows the comparison of the number of coughs in individual animals in
both groups. There was a significant interaction between the antioxidant
treatment and hyperoxia (two-way ANOVA, P=0.004). |
Effects of supplementation with vitamins C and E on mechanically-induced cough in guinea-pigs exposed to 100% O2
There were no significant differences among the two experimental and control
groups of animals in mechanically-induced cough from LPh mucosa. However, we
determined a significant increase in the mechanically-induced number of coughs
from TBr region in the experimental T-H (antioxidant-hyperoxic) group, after
exposures to hyperoxia, compared with H (hyperoxic alone) animals [2.5(1-4)
vs. 1.0(1-2); P<0.01] (
Fig. 2A). There also was a modest but significant,
decrease in the mechanically-induced TBr cough between the air breathing control
group and the H group [2.0(1-3)
vs. 1.0(1-2); P<0.05] (
Fig. 2A).
Hyperoxic exposure attenuated the intensity of cough bouts expressed in kPa
and lessened the data spread, as judged from a smaller SD, compared with the
normoxic control, but these changes did not assume statistical significance
(
Fig. 2B). At the same time, the results revealed a significant increase
in the intensity of cough bouts in the T-H animals, compared with the H animals
[8.9(4.2)
vs. 5(2.5); P<0.05] (
Fig. 2B).
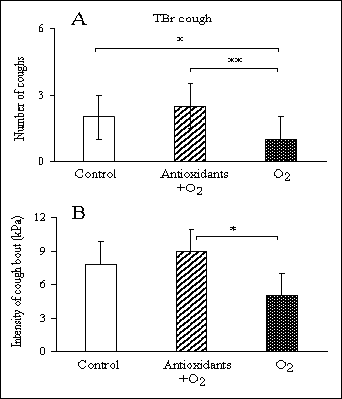 |
Fig. 2. Changes in mechanically-induced tracheobronchial (TBr) cough expressed as the number of coughs (panel A) and the intensity of cough bouts (panel B) in the control group (air breathing), the antioxidant-hyperoxia group, and the hyperoxia-alone group. The number of coughs is expressed as median and interquartile range and the intensity of cough bouts as means ±SD. *P<0.05, **P<0.01. |
Histology
Histological examination of specimens taken from the tracheobronchial tree of hyperoxic animals revealed some pathological changes including hyperplasia of the epithelium, dilatation of mucosal lymphatic and blood vessels, with the appearance of lymphocytes, eosinophils, and neutrophils. More intense histopathological changes were seen in lung tissue.
There was emphysematous infiltration with non-specific inflammatory cells, characteristic of pneumonitis, and a secondary reduction of alveolar spaces. Other changes included diffused excessive vascular dilatation, congestion, oedema, and inflammation, with aggregates of lymphocytes (not shown). We failed to recognize any appreciable differences in the intensity and quality of morphological changes between the animals of T-H group (antioxidant-hyperoxia) and H group (hyperoxia alone).
DISCUSSION
Hyperoxia-induced oxidative stress is well characterized in rodents and has been used as a valuable model of the human respiratory distress syndrome (11, 19, 20). The susceptibility of the lung to oxidative injury depends largely on its ability to upregulate protective ROS scavenging systems when exposed to oxidative stress such hyperoxia (21). Many recent studies demonstrate that antioxidants, by virtue of antiinflammatory effects protect the lung in a model of oxidative lung injury (11, 19, 20). The relationship between airway oxidative damage and supplementation of antioxidant substances is still unclear and many findings are controversial (22, 23).
To expand on our preliminary studies that revealed the inhibitory effect of hyperoxia on cough, taken as an index of airway dysfunction (7-9), in this study we used a model of oxidative stress consisting of exposure of guinea-pigs to high oxygen level. The goal was to test the assumption that augmentation of antioxidant capacity by exogenous substitution might minimize the airway damage by reducing ROS produced by oxygen, which would be reflected in changed cough reaction. We chose vitamin C and vitamin E supplementation as representative of action in the water and lipid cellular phases, respectively, in doses suggested in the literature (10, 24).
The results of this study showed an evident tendency to a decrease in chemically-induced cough after exposure to hyperoxia, although this effect was somewhat less than that shown in previous reports (7, 8, 9). Therefore, the intensity of hyperoxia-induced airway inflammation was strong enough to affect nerve endings in the airways responsible for mediation and modulation of cough. Histological findings are in accordance with this supposition, because we found signs of injury to airway mucosa and lung tissue in hyperoxic animals. Toxic effects of high oxygen breathing on lung function and structure are known (3, 4, 5). There is increasing evidence that oxidative stress is implicated in the development of airway inflammation induced by hyperoxia. Hyperoxia-induced lung injury can be considered a bimodal process resulting from direct oxygen toxicity and from the accumulation of inflammatory mediators in the lungs. Both apoptosis and necrosis have been described in alveolar cells during hyperoxia (11). Studies related to the interactions "hyperoxia - cough reflex" and "antioxidants - hyperoxia - cough reflex" are limited. Our previous work has shown the inhibitory effect of hyperoxia on cough (7-9). Morphological changes accompanying hyperoxia, manifesting in airway and lung damage, can contribute to decreased cough. ROS overproduction is liable to be involved in cough attenuation by hyperoxia. At the present state of knowledge, however, it is unknown what level of ROS or any other components of oxidative stress attenuates the intensity of cough. That oxidative stress is at play in the hyperoxia-induced cough attenuation may be inferred from the reversal of this effect on the background of antioxidant vitamin supplementation observed in the present study.
The oxygen-induced airway inflammation seems to affect sensory afferent nerve endings in lower rather than upper airways. This is evidenced by the mechanical stimulation experiment of the present study in which antioxidant supplementation disinhibited hyperoxia-evoked cough attenuation in response to tracheobronchial but not laryngopharyngeal stimulation. Likewise, histological observation point to marked pathological changes in small airways and lung tissue and Putman et al (25) reported different levels of antioxidants in upper and lower airways. Oxidants interact primarily with the epithelial lining fluid layer. This layer is thick and contains high levels of antioxidants in upper airways. Therefore, oxidant injury in this area is rare and it is more common in lower airways, where the epithelial lining fluid layer is thinner.
In this study, we used two different methods to elicit cough: chemical and mechanical stimulation. While chemically induced cough was evoked in awake animals, mechanical stimulation was performed in anesthetized ones. Another difference between the two methods was that citric acid aerosol (chemical) affected the whole surface of airways, while mechanical stimulation was restricted to confined areas. Although antioxidants did not cause any obvious changes in the morphological picture, they increased cough, inhibited by hyperoxia, on both chemical and mechanical stimulation, the later in the tracheobronchial region only.
Many studies describe a protective effect of antioxidants reflecting a reduction in oxidative stress (10-13). On the other hand, although oxidative stress is implicated in the etiology of diseases, most supplementation trials with antioxidant micronutrients have not shown the expected beneficial effects (26). There is no evidence of synergistic or cooperative interaction between vitamin C and E on DNA damage or lipid peroxidation in people with oxidative challenge. Similarly, Langley et al (27) reported that vitamin E is unable to protect the lungs of guinea-pigs from ROS in the absence of a sufficient primary enzymatic antioxidant defense (27). Other randomized controlled trials reported no favorable effect of vitamin E supplementation on the incidence and severity of acute respiratory tract infection in elderly people (28).
In conclusion, our results indicate a protective effect of antioxidant supplementation on the oxidant-mediated cough depression. Thus, the use of antioxidants during oxygen therapy has the potential to uphold the strength of the defensive cough reflex, which may be of significance in respiratory infections.
Acknowledgments:
The authors wish to thank Lila Surinova, Lenka Mazurova, Tomas Zatko, Jana Calikova,
and Miroslav Vrabec for their outstanding technical assistance. This study was
supported by National Research Grant VEGA 1/2277/05.
REFERENCES
- Chen B, Guber A, Marom Z. Advance long-term oxygen therapy. RT International 1995: 55-60.
- Tarpy SP, Celli BR. Long-term oxygen therapy. N Eng J Med 1995; 14: 710-714.
- Jenkinson S. Oxygen toxicity. New Horizons 1993; 1: 47-57.
- Clark JM, Lambertsen CJ. Pulmonary oxygen toxicity: a review. Pharmacol Rev 1971; 23: 37-132
- Klein J. Normobaric pulmonary oxygen toxicity. Anesth Analg 1990; 70: 195-207.
- Capellier G, Maupoil V, Boussat S, Laurent E, Neidhardt A. Oxygen toxicity and tolerance. Minerva Anesthesiol 1999; 65: 388-392.
- Brozmanova M, Hanacek J, Tatar M, Karcolova D, Szepe P. The influence of pure normobaric oxygen breathing for 48 h and 60 h on respiratory reflexes in guinea pigs. Eur Resp J 1996; 9(Suppl 23): 131.
- Brozmanova M, Hanacek J, Tatar M, Kollarik M. The influence of hyperoxia on cough reflex intensity in guinea pigs treated with bleomycin. Bratisl Lek Listy 2004; 105: 65-71.
- Brozmanova M, Hanacek J, Tatar M, Strapkova A, Szepe P. Effects of hyperoxia and allergic inflammation on cough reflex intensity in guinea pigs. Physiol Res 2002; 51: 529-536.
- Cadenas S, Rojas C, Perez-Campo R, Lopez-Torres M, Barja G. Vitamin E protects guinea pig liver from lipid peroxidation without depressing levels of antioxidants. Int J Biochem Cell Biol 1995; 27: 1175-1181.
- Pagano A, Barazzone-Argiroffo C. Alveolar cell death in hyperoxia-induced lung injury. Ann NY Acad Sci 2003; 1010: 405-416.
- MacNee W. Oxidants/antioxidants and COPD. Chest 2000; 117: 303-317.
- Hubbard R, Fogarty A. The developing story of antioxidants and asthma. Thorax 2004; 59: 3-4.
- Jacob RA. The integrate antioxidant system. Nutr Res 1995; 15: 755-766
- Lambelet P, Sancy F, Lolliger J. Chemical evidence for interactions between vitamin E and C. Experentia 1985; 41: 13384-13388.
- Xiang A, Uchida Y, Nomura A et al. Effects of airway inflammation on cough response in the guinea pig. J Appl Phys 1998; 85: 1847-1854
- Korpáš J, Bilèík P, Kohút A. Tracheal canulla for chronic experiments. Ès Fyziol 1964; 13: 482-483 (in Slovak).
- Korpas J, Tomori Z. Cough and Other Respiratory Reflexes. Basel, Karger, 1979, p. 356.
- Jyonouchi H, Sun S, Abiru T, Chareancholvanich S, Ingbar DH. The effects of hyperoxic injury and antioxidant vitamins on death and proliferation of human small airway epithelial cells. Am J Respir Cell Mol Biol 1998; 19: 426-436.
- Abraham NG. Hem oxygenase in biology and medicine 2002, p. 516.
- Comhair SAA, Erzurum SC. Antioxidant responses to oxidant-mediated lung diseases. Am J Physiol Cell Mol Physiol 2002; 283: 246-255.
- Oldham KM, Bowen PE. Oxidative stress in critical care: is antioxidant supplementation beneficial? Am Diet Assoc 1998; 98: 1001-1008.
- Rahman I. Oxidative stress and gene transcription in asthma and chronic obstructive pulmonary disease: antioxidant therapeutic targets. Curr Drug Targets Inflamm Allergy 2002; 1: 291-315.
- Cadenas S, Rojas, C, Barja G. Endotoxin increases oxidative injury to proteins in guinea pig liver: Protection by dietary vitamin C. Pharmacol Toxicol 1998; 82: 11-18.
- Putman E, van Golde LM, Haagsman HP. Toxic oxidant species and their impact on the pulmonary surfactant system. Lung 1997; 175: 75-103.
- Choi SW, Benzie IF, Collins AR, Hannigan BM, Strain JJ. Vitamins C and E: acute interactive effects on biomarkers of antioxidant defence and oxidative stress. Mutat Res 2004; 551: 109-117.
- Langley SC, Phillips GJ, Tahedl S, Kelly FJ. Dietary supplementation of vitamin E fails to prevent the development of hyperoxic lung injury in the premature guinea pigs. Comp Biochem Physiol Comp Physiol 1992; 103: 793-799.
- Graat JM, Schouten EG, Kok FJ. Effect of daily vitamin E and multivitamin-mineral supplementation on acute respiratory tract infections in elderly persons. JAMA 2002; 288: 715-721.