Cholestasis is characterized by an abnormal accumulation of bile acids and by defect in the process of bile acid transport leading to impairment in bile formation. It is a common condition in human liver diseases due mainly to the obstruction of the bile ducts and results in progressive liver injury culminating in cirrhosis and liver failure (1). Unfortunately, the therapeutic options for treating this syndrome remain limited, because, in part, the essential mechanisms mediating cholestatic liver injury are still incompletely understood (2). The mechanisms triggered by cholestasis are yet to be revealed, but some key factors have been highlighted in recent studies: cell death either by necrosis or apoptosis, a disruption in the oxidative stress balance, an inflammatory response, the release of profibrotic cytokines leading to the activation of myofibroblasts and the modification in the extracellular matrix (ECM), but also bile duct epithelial cell proliferation (3, 4). Altogether, these mechanisms result in the development of liver fibrosis.
Bile duct ligation (BDL) is an experimental model in rats characterized by rapidly progressing biliary fibrosis. The initial stages are represented by acute cholestasis, in which oxidative stress and inflammation play essential roles. There is an increase generation of reactive oxygene species (ROS) and a depletion of the antioxidant capacity of the liver tissue. It seems that the oxidative stress may not be the primary aggressor but rather the consequence of inflammation and bile acids accumulation (5).
Statins are cholesterol-lowering agents that inhibit 3-hydroxy-3-methyl-glutaryl coenzyme A (HMG-CoA) reductase, a key enzyme that catalyzes the rate-limiting step within the cholesterol biosynthetic pathway. Statins were shown to exert antiinflammatory effects by several ways: inhibition of cytokine formation and adhesion molecules, reduction of nitric oxide production (6). They inhibit the proliferation of fibroblasts and protein synthesis and also reduce the generation of profibrotic cytokines in extrahepatic tissue (7). They are also capable to reduce the portal hypertension (8). It seems that statins have a beneficial role on the pathogenic pathways involved in liver fibrosis by decreasing the activation of hepatic stellate cells (HSCs) and lowering the level of collagen (9).
Among the statins, rosuvastatin (Ro) seems to be a potential candidate for the
treatment of cholestasis in humans. Ro is a HMG-CoA reductase inhibitor with
a more potent affinity for the active site of HMG-CoA reductase than other statins.
It is thought that Ro is also distributed principally to the liver in humans,
as shown by its high proportion (>70%) of nonrenal clearance (10). Awad
et
al. have administered Ro 10 mg/bw for 7 days to bile duct ligated rats starting
from the 3
rd day after BDL and have showed that
it improved biliary obstruction induced-injury and reduced oxidative stress
and inflammation in the liver (11).
Despite those beneficial effects, recent studies have shown that statins may exert a negative effect on the liver parenchyma when administered in bile duct ligated rats by increasing the levels of alanine aminotransferase (ALT) and aspartate aminotransferase (AST) (9), by lowering the antioxidant capacity of the liver and by creating mitochondrial disfunction (5).
Our study aimed to investigate the systemic effects of different doses of Ro
on a model of induced cholestasis in rats. We explored i) the influence of Ro
on oxidative stress parameters in liver, plasma, kidney and brain ii) for the
liver tissue, we correlated the oxidative stress parameters with the early changes
regarding inflammation, necrosis, fibrosis, bile duct proliferation and the
level of alfa smooth muscle actine (a-SMA), proliferating cell nuclear antigen
(PCNA), transforming growth factor beta-1 (TGF-ß1) and nuclear factor

B (NF-

B).
It could be a crucial step in order to establish the potential medical use of
Ro in cholestatic liver diseases.
MATERIALS AND METHODS
Experimental design
A total of 40 female Wistar rats, weighing 253±21.2 g, three months old, were
used in this study. The animals were randomly divided into 4 groups (n=10).
Group 1 (Sham) underwent laparotomy alone and the common bile duct was only
dissected from the surrounding tissue, and no drug was applied. Group 2 (BDL)
was subjected only to bile duct ligation (BDL). Group 3 (Ro 5) received a daily
dose of 5 mg/bw Ro orogastric starting 24 hours after BDL. Group 4 (Ro 10) received
a daily dose of 10 mg/bw Ro orogastric starting 24 hours after BDL. Wistar albino
rats were obtained from the Animal Department of “Iuliu Hatieganu” University
of Medicine and Pharmacy from Cluj-Napoca. They were kept for ten days with
12 h dark and 12 h light regimen in the Physiology Department in order to acclimatize,
at room temperature (22°C). Animals were fed with a standard pellet diet and
received water
ad libitum. All the experiments were performed according
to the approved animal-care protocols of the Ethical Committee on Animal Welfare
of the “Iuliu Hatieganu” University in accordance with the Romanian Ministry
of Health and complying with the Guiding Principles in the Use of Animals in
Toxicology.
Bile duct ligation
The bile duct ligation was performed as previously described (4). In short, each rat was anesthetized using ketamine xylazine cocktail (90 mg/bw ketamine, 10 mg/bw xylazine). The abdomen was shaved and disinfected with 10% povidone iodine, a midline laparotomy was performed. The common bile duct was isolated and ligated with 4–0 silk suture. The rats were then allowed to recover with free access to chow and water. In day 7 from the beginning of the experiment, each group of animals was sacrificed with sodium pentobarbital (60 mg/rat ip). Blood, liver, brain and kidneys were collected.
Preparation of biological samples
All the animals were weighted at the beginning and at the end of the experiment, as well as the collected livers. The left lobe of the liver was immersed in 10% formalin solution and prepared for histological analysis. The rest of the liver, the brain and kidneys were washed with cold saline and homogenized with a Polytron homogenizer (Brinkman Kinematica, Switzerland) in 50 mM TRIS–10 mM EDTA buffer (pH 7.4). The suspension was centrifuged for 5 min at 3,000 g and 4°C to prepare the cytosol fraction. Plasma as well as supernates from each animal was stored in aliquots at –80°C until assayed.
Measurement of oxidative stress parameters
The protein levels in homogenates were measured with the Bradford method (12). Malondialdehyde (MDA) was determined using the fluorimetric method with 2-thiobarbituric acid described by Conti (13). The MDA was spectrofluorimetrically determined in the organic phase using a synchronous technique with excitation at 534 nm and emission at 548 nm.
Protein carbonyls (PC) were determined using the fluorimetric method with 2,4-dinitrophenyl-hydrazine (14). The readings were performed using a spectrophotometer at 355–390 nm and to calculate the remaining carbonyl fragments the molar extinction coefficient with a value of 22,000/M/cm was used.
Reduced glutathione (GSH) and oxidized glutathione (GSSG) were measured fluorimetrically using o-phtalaldehyde. The fluorescence was recorded (350 nm excitation and 420 nm emission) and the concentrations for GSH and GSSG were determined using standard curves (15, 16).
Liver function
To determine the liver function we assessed the serum activity of aspartate aminotransferase (AST), alanine aminotransferase (ALT) and gamma-glutamiltransferase (GGT), as well as the bilirubin plasma level (BT) by semiautomatic analysis, using colorimetric assay kits, according to the manufacturer’s instructions (17).
Histological examination
Samples were embedded in paraffin. The sections were made at 4 micrometers with a microtome Leica RM 2125 RT and stained by haematoxiline-eosine (HE) and Masson’s trichrome methods. Then the slides were examined under a microscope Olympus BX 51.
The images were taken with Olympus DP 25 digital camera and processed by a special image acquisition and processing program: Olympus Cell B. Sections were examined by an independent observer blinded to the experimental protocol. The grade of necro-inflammation was assessed using a histologic grading system adapted from Knodell Histology and Activity Index (18).
The stage of fibrosis was assessed using the following criteria: (0 – absence; 1 – portal fibrosis; 2 – portal fibrosis and few septa; 3 – evident septal fibrosis without cirrhosis; 4 – cirrhosis). The number of biliary canals in five portal sites for each section was also noted.
Immunohistochemical study
Immunohistochemical study was realized using antibodies against Alfa Smooth
Muscle Actin (dilution 1/200, ab 5694, Abcam, Cambridge, UK), against Proliferating
Cell Nuclear Antigen (Clone PC 10, dilution 1/300, Dako Cytomation, Glostrup,
Denmark), against TNF-

receptor II (polyclonal rabbit anti-rat and human TNF receptor II, ab15563,
Abcam, Cambridge, UK) and against CD 68 (LINARIS Biologische Produkte, Dossenheim,
Germany, dilution 1/150).
For immunohistochemistry, sections were dewaxed and rehydrated, heat-mediated epitope retrieval was realized by immersion of samples in boiling citrate buffer pH 6, using a pressure-cocker. Sections were cooled in citrate buffer at room temperature and washed in phosphate buffered saline (PBS). Endogenous peroxidase activity was blocked using 3% hydrogen peroxide in methanol for 10 minutes. Sections were incubated overnight with the primary antibodies. Secondary reaction was realized using Novostain Universal Detection kit (Novocastra, Newcastle, UK). Positive reaction was visualized using 3, 3’-diamino-benzidine (DAB). Sections were counterstaind with Gill 2 haematoxylin, dehydrated and mounted.
The numbers of PCNA positive cholangiocytes were assessed by counting 500 cells in non-overlapping fields for each slide and the data expressed as percentage of positive cells. In the liver parenchyma hepatocytes from five non-overlaping high power fields were counted and results expressed as percentage of PCNA positive cells.
The anti-smooth muscle actin antibody was used for myofibroblast identification.
The quantification of TNF-

receptor II (TNFR2) expression was carried out after a protocol previously described
(19). The quantification was carried out visually by counting the positive cells
in 10 high power fields/slide at the 40x objective amplification, following
the next semi quantitative scale: score 0 (“basically no staining”) was given
for positive immunohistochemical staining for less than 5% of the cells; score
1 for 5–25% (“weak”) positive staining; score 2 (“moderate”) for 26–50% positive
staining and score 3 (“strong”) for more than 50% positive staining. The mean
values were calculated and compared between experimental groups.
Immunofluorescence examination
TGF-ß1 expression was assessed using laser scanning confocal microscopy. Anti TGF-ß1 antibody was purchased from Abcam (ab27969, Cambridge, UK). For detection, a goat polyclonal secondary antibody to mouse IgG conjugated with tetramethylrhodamine isothiocyanate (TRITC) (ab 6786, Abcam, Cambridge, UK) was used. The nuclei were counterstained with Draq 5 (4084 S, Cell Signaling Technology, Massachusetts).
Fluorescent images were acquired using a Zeiss LSM 710 confocal laser scanning unit (Oberkochen, Germany) equipped with argon and a HeNe laser mounted on an Axio Observer Z1 Inverted Microscope. Cryosections were made and mounted on poly-L-lysine coated slides. Sections were fixed in ice-cold acetone. After three washing procedures in PBS for 15 min, the slides were covered for 10 min with a protein blocking buffer (Novocastra, Newcastle, UK).
The dying procedures were made in accordance with manufacturers’ protocols. Specific visualization of cell structures was performed using 543 nm and 633 nm excitation laser lines to detect Draq5 (661–757 nm emission) and TRITC (547–630 nm emission), respectively. We used the following Beam Splitters: MBS 488/543/633.
Western blot assay
Samples of snap-frozen livers were homogenized in lysis buffer containing Igepal-nonidet 1% (Sigma), 1% protease inhibitor complex (Sigma) in PBS for 1 hour, on ice. Cell extracts were spun at 14,000 g for 30 min at 4°C. Supernatant was collected and 50 µl were used to determinine the protein content by the Bradford method (Biorad, USA). Lysates were mixed 1:2 (v/v) with Laemli sample buffer (BioRad, Hercules, CA, USA) containing 2-mercaptoethanol and the proteins were denaturized at 95°C for 10 minutes. Samples (20 µg of protein/lane) were subjected to SDS–PAGE (12% polyacrylamide) at 200 mV, and proteins were blotted on polyvinylidenedifluoride membranes (BioRad,USA) for 60 min at 100 mV, using Biorad Miniprotean system (BioRad). Blots were blocked in 5% nonfat dry milk in PBS, containing 0.1% Tween 20 (PBS-T) for 1 hour at room temperature, incubated with the primary antibody (1:1000) for a-SMA (Abcam plc, Cambridge, UK), NF-

B, pNF-

B or GAPDH (Santa Cruz Biotechnology, Santa Cruz, CA, USA). Thereafter, the membranes were incubated with corresponding secondary peroxidase-coupled antibody (1:1500) (Santa Cruz Biotechnology, Santa Cruz, CA, USA). GAPDH served as endogenous control. Proteins were detected using Supersignal West Femto Chemiluminiscent substrate (Thermo Fisher Scientific, Rockford IL, USA) and the membranes exposed to an X-ray film (Kodak) for approximately 2 min. The films were developed and analyzed using Phoretix array (free trial version). Western blot analyses from all groups were calibrated to sham-operated rats set to 100%.
Statistical analysis
Experimental data were analyzed by one-way analysis of variance (ANOVA) followed by the Tukey´s multiple comparisons posttest using GraphPad Prism 5.0 software (GraphPad, San Diego, Ca., SUA). The data were expressed as means ± standard de
viation (S.D.). A p<0.05 was considered statistically significant. In the graphs we only marked the values that were significantly modified compared to the BDL group.
RESULTS
All the animals survived until the end of the experiment. All animals with bile duct ligation had jaundiced after BDL; they presented weight loss (BDL group: 253±21.2 g, Ro 5: 234±18.7 g, Ro 10: 241±23.84 g). A slight increase in the weight of the liver was found in the rats that underwent BDL (sham: 10±0.86 g, BDL: 12±0.79 g, Ro 5: 12±0.48 g, Ro 10: 13±1.7 g).
Biochemical parameters
The values of biochemical measurements for the different groups are shown in
Table 1. AST and ALT serum levels are considered to be markers of hepatocyte
destruction. AST and ALT were significantly increased in the BDL group in comparison
to the sham operated group (p<0.01). AST and ALT values were increased additionally
by the administration of Ro 5 mg and 10 mg as compared to the BDL group (p<0.01).
Table 1. Comparative
plasma biochemical measurements at 7 days from the beginning of the study
(mean ±S.D.). 24 hours after BDL, the Ro 5 and Ro 10 groups have
received daily 5 mg Ro/bw, and 10 mg Ro /bw, respectively. |
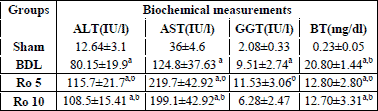 |
a p<0.01 vs.
Sham, b p<0.05 vs. BDL. |
The levels of GGT and BT are commonly used in patients to evaluate cholestasis. GGT was increased in the BDL group compared to the sham group (p<0.05). The administration of Ro didn’t influence significantly the level of GGT in the serum, but we can observe a tendency towards the reduction of this parameter when 10 mg of Ro were administered after bile duct ligation. BT levels were increased in the BDL group as compared to Sham (p<0.001). The administration of Ro 5 mg and Ro 10 mg decreased BT levels as compared to the BDL group, p<0.001.
Oxidative stress parameters
To evaluate the presence of oxidative stress in the liver, brain, kidney and serum we used indirect methods. In our study we evaluated the products resulted from the oxidation of lipids by determining the malondialdehyde (MDA) and also the effects on proteins by determining protein carbonyls (PC). We also evaluated the antioxidant capacity by determining reduced glutathione (GSH)/oxidized glutathione ratio (GSSG).
In the liver, the levels of MDA were significantly higher in the group that
underwent BDL as compared to the Sham group (0.10±0.01 nmoles/mg protein BDL
group vs. 0.06±0.02 nmoles/mg protein Sham, p<0.05). When Ro was administered
in either dose, it significantly increased MDA as compared to Sham group (0.13±0.03
nmoles/mg protein Ro 5; 0.15±0.03 nmoles/mg protein Ro 10
vs. 0.06±0.02
nmoles/mg protein Sham group, p<0.05). Moreover, the dose of 10 mg/bw increased
MDA significantly as compared to the group that underwent only bile duct ligation
(
Table 2).
Table 2. The levels
of oxidative stress parameters in the liver, plasma, brain, and kidney,
7 days from the beginning of the study (the values are expressed as mean±S.D.).
24 hours after BDL, the Ro 5 and Ro 10 groups have received daily 5 mg
Ro/bw, and 10 mg Ro /bw, respectively. |
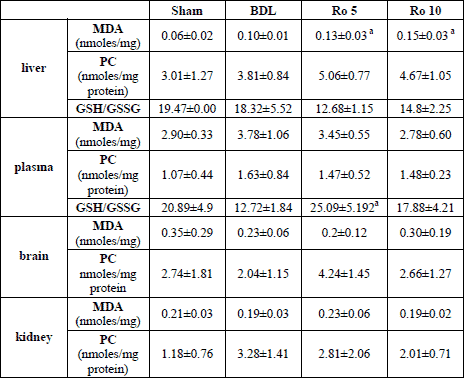 |
a p<0.05 vs.
BDL. |
The protein carbonyls were significantly increased by the administration of
Ro as compared to the Sham group (5.06±0.77 nmoles/mg protein Ro 5; 4.67±1.05
nmoles/mg protein Ro 10 vs. 3.01±1.27 nmoles/mg protein Sham group p<0.05).
BDL didn’t determine a significant increase in the levels of PC in the liver
(
Table 2).
The antioxidant capacity was quantified using the reduced glutathione (GSH)/oxidized
glutathione ratio (GSSG) the liver. This ratio (
Table 2) was not modified
by the ligation of the bile duct, or by the administration of Ro.
In the plasma, the levels of MDA and PC weren’t influenced by BDL or by Ro. The reduced glutathione (GSH)/ oxidized glutathione ratio (GSSG) was significantly decreased by the administration of rosuvastatin 5 mg.
In the brain and kidney the same markers of oxidative stress were quantified.
Since no important modifications were found their values are listed in
Table
2.
Inflammation assessment
The modulation of inflammation in the liver by bile duct ligation and the administration of Ro was evaluated:
1) histologically, using the necro-inflammatory score; 2) immunohistochemically,
using specific staining for TNFR2 and Kuppfer cells, respectively; 3) by Western
blot, assessing the induction of NF-

B
and activation with pNF-

B.
The necro-inflammation score (
Table 3) was augmented by BDL, and the
administration of Ro in both doses didn’t improve it, more so we could observe
a tendency towards a higher score in the groups that received the statin. Liver
sections (
Fig. 1) from Sham operated rats showed normal histology. Rats
from BDL group showed large foci of hepatic parenchyma necrosis with marked
inflammatory cell infiltration. In the Ro10 group large foci of necrosis with
marked inflammation were observed (
Fig. 1).
Table 3. Scores for
necro-inflammation and fibrosis using Knodell HAI grading system and number
of biliary ducts (mean±S.D.). The parameters were assayed 7 days
after the beginning of the experiment. The groups with Ro have received
daily 5 mg Ro/bw (Ro 5), respectively 10 mg Ro/bw (Ro 10), beginning 24
hours after the BDL. |
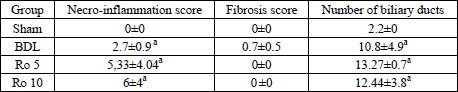 |
a p<0.01 vs.
Sham. |
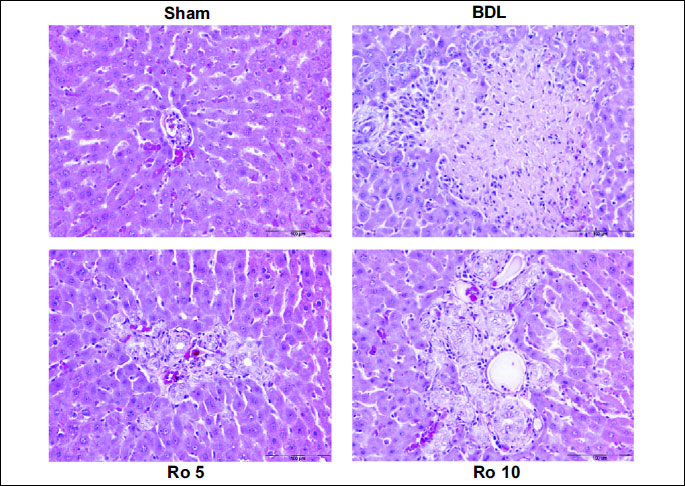 |
Fig.
1. Haematoxylin-eosin stain showing the effects of 6 days treatment
with rosuvastatin (Ro 5: 5 mg/bw rosuvastatin orogastric; Ro 10: 10 mg/bw
rosuvastatin orogastric) after bile duct ligation (BDL) in Wistar rats
on the liver parenchyma. Representative photomicrographs of haematoxylin-eosin
statin from each treatment group with original magnification of 200x,
Scale bar=100 µm. Sham operated group showing normal liver
histology; BDL group showing large area of parenchimal necrosis
and inflammatory cell infiltration; Ro 5 group typical ductular
reaction, slight inflammatory infiltrate; Ro 10 group ductular
reaction, inflammatory cell infiltration. |
For TNFR2 (
Fig. 2A), sections from the Sham operated group showed no
or occasionally weak TNFR2 expression. Positive cells were randomly distributed
within hepatic lobules. Animals from the experimental groups (BDL, Ro 5 and
Ro 10) showed a significant upregulation of the TNFR2 expression. Most of the
TNFR2 positive hepatocytes were localized in the central lobular and midzonal
areas. The staining was cytoplasmic and intense.
Within experimental groups, the highest level of TNFR2 expression was found
in the Ro 10 group, the average score being 2.33±0.36 as compared to the BDL
group (0.83±0.04) (
Fig. 2B).
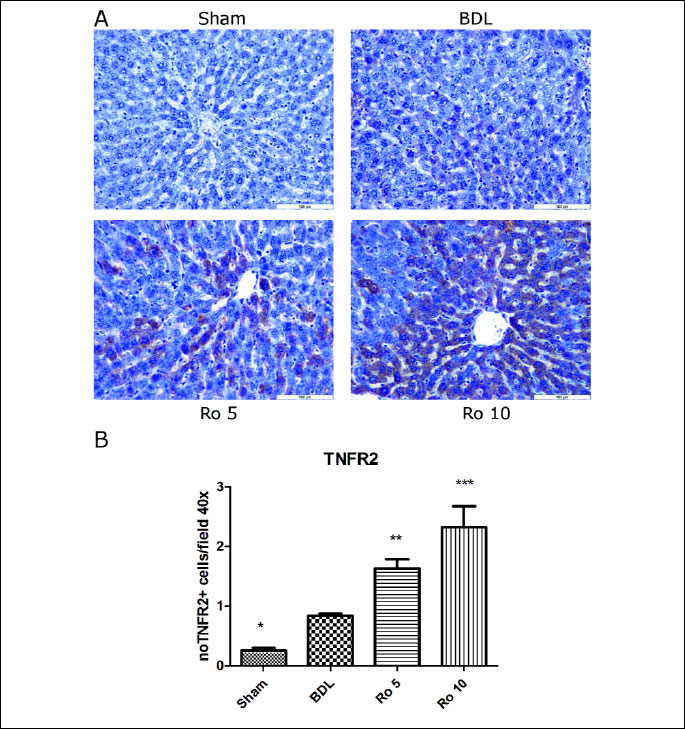 |
Fig.
2. TNFR2 expression in liver parenchima of Wistar rats that were treated
6 days with rosuvastatin (Ro 5: 5 mg/bw rosuvastatin orogastric; Ro 10:
10 mg/bw rosuvastatin orogastric) after bile duct ligation (BDL). (A)
Immunoperoxidase technique counterstaind with Gill 2 hematoxylin Bar=100
µm. (B) The quantification was carried out visually by counting
the positive cells in 10 high power fields/slide at the 40x objective
amplification, following the next semi quantitative scale: score 0 (basically
no staining) was given for positive immunohistochemical staining
for less than 5% of the cells; score 1 for 525% (weak)
positive staining; score 2 (moderate) for 2650% positive
staining and score 3 (strong) for more than 50% positive staining.
The data were analyzed by one-way ANOVA followed by the Tukey´s
multiple comparisons posttest using GraphPad Prism 5.0 software (GraphPad,
San Diego, SUA). The data were expressed as means ±S.D.;*p<0.05,
**p<0.01, ***p<0.001 vs. BDL. |
The Kuppfer cells (
Fig. 3A) or CD68-positive cells were quantified on
8 fields of X40 sections using an Olympus BX51 light microscope and an increased
number of Kuppfer cells were found in the liver of the rats that underwent bile
duct ligation as compared to the Sham group (p<0.001). The administration of
5 mg of Ro further augmented significantly the number of CD 68-positive cells
compared to the BDL group (p<0.01). To the contrary, a larger quantity of statin
(10 mg Ro) lowered the number of Kuppfer cells significantly (
Fig. 3B)
as compared to the BDL group (p<0.01).
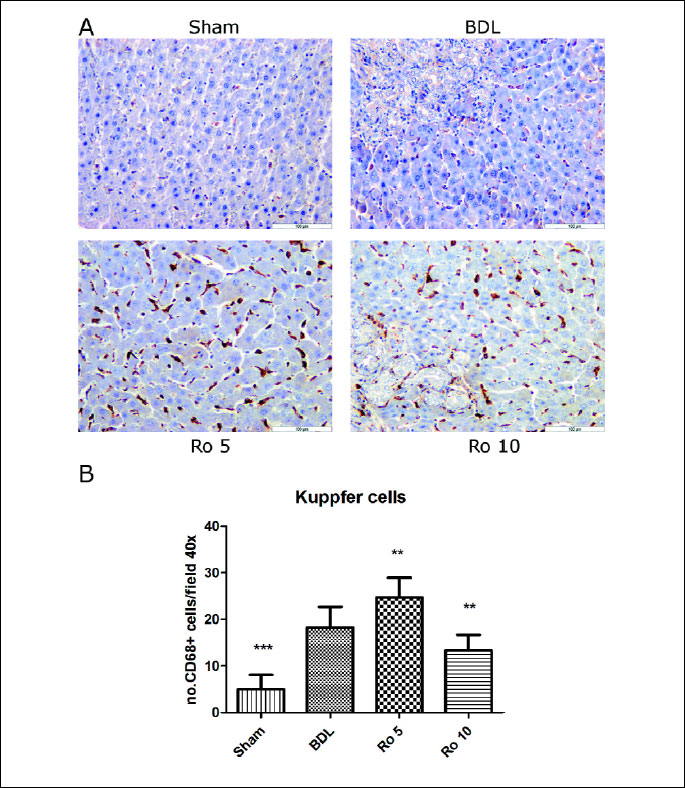 |
Fig.
3. CD68 expression in liver parenchima of Wistar rats that were treated
6 days with rosuvastatin (Ro 5: 5 mg/bw rosuvastatin orogastric; Ro 10:
10 mg/bw rosuvastatin orogastric) after bile duct ligation (BDL). (A)
Immunoperoxidase technique counterstained with Gill 2 hematoxylin Bar=100
µm. (B) CD68-positive cells were quantified on 8 fields of
x40 sections using an Olympus BX51 light microscope. The data were analyzed
by one-way ANOVA followed by the Tukey´ s multiple comparisons posttest
using GraphPad Prism 5.0 software (GraphPad, San Diego, SUA). The data
were expressed as means ±S.D.;**p<0.01, ***p<0.001 vs.
BDL. |
The induction and activation of NF-

B
were quantified by western blot (
Fig. 4A). Using this method we didn’t
find any significant difference between the Sham operated group and the BDL
group regarding the induction (
Fig. 4B) and activation of NF-

B
(
Fig. 4C). The administration of Ro in both doses significantly reduced
NF-

B levels in
the cytoplasm and also decreased the activation of NF-

B
(reduced levels of the phosphorylated form, pNF-

B).
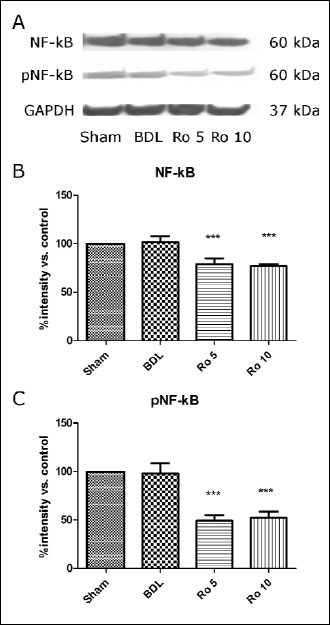 |
Fig. 4. Effects of prophylactic
treatment with rosuvastatin on induction and activation of Nf- B,
assessed by Western blot analysis of NF- B
and pNF- B
expression in the rat liver. The rats were treated for 6 days starting
1 day after BDL or were left untreated for the corresponding period, (each
group with a minimum of n=10). Representative Western blots (NF- B
and pNF- B
and endogenous control GAPDH) and quantifications are shown (means ±S.D.;
data are compared to sham-operated rats, which are set to 100%), ***p<0.001
vs. BDL. |
Liver fibrosis assessment
Liver fibrosis was evaluated using histological methods (Masson’s trichrome
and a fibrosis score), immunohistochemically (staining for

-SMA),
confocal microscopy for TGF-ß1 and western blot for

-SMA.
This experiment being focused on the incipient phases of cholestasis and liver
injury in the experimental groups, no portal fibrosis was found and also no
obvious differences between experimental groups regarding the results of Masson’s
trichrome staining (
Fig. 5) and a fibrosis score (
Table 3).
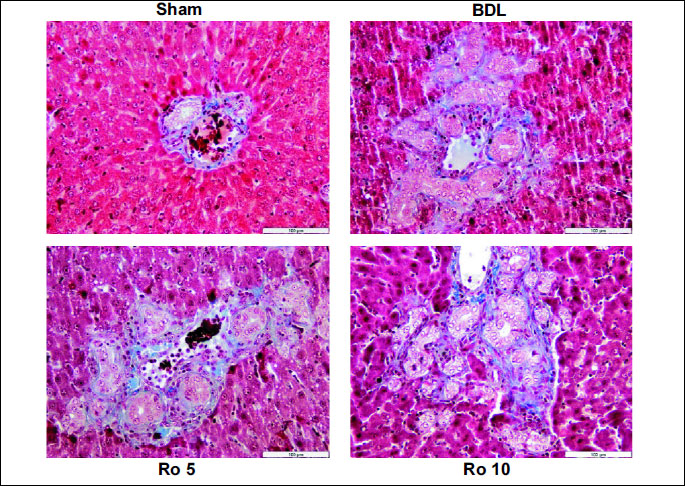 |
Fig.
5. Massons trichrome stain used to evaluate the effects of the
6 days treatment with Rosuvastatin (Ro 5: 5 mg/bw rosuvastatin orogastric;
Ro 10: 10 mg/bw rosuvastatin orogastric) after bile duct ligation (BDL)
in Wistar rats on the liver parenchyma. Representative photomicrographs
of Massons trichrome stain from each treatment group with original
magnification of 200x, Scale bar=100 µm. Sham operated group
showing normal liver histology; BDL, Ro 5, Ro 10:
no portal fibrosis, no obvious differences between experimental groups. |
For the

-SMA immunoreactivity,
in the Sham operated group, immunohistochemically,

-SMA-positive
cells were observed in the tunica media of blood vessels in the portal areas.
In the BDL, Ro 5 and Ro 10 groups, numerous

-SMA-positive
cells were observed around the proliferating bile ducts in the portal areas
(
Fig. 6A). No significant differences between these groups were observed.
However, increased levels of

-SMA
(
Figs. 6B, 6C) were detected in the liver lysates of rats from the BDL
group using Western blot (p<0.001). The administration of 10 mg rosuvastatin
decreased

-SMA
(p<0.01).
 |
Fig.
6. Effects of prophylactic treatment with rosuvastatin on hepatic
myofibroblast accumulation, assessed by (A) immunohistochemistry
for a-SMA of cryo-liver sections and (B) Western blot analysis
of hepatic alpha smooth muscle actin ( -SMA)
expression. The rats were treated with Ro 5 mg/bw or 10 mg/bw for 6 days
starting 1 day after BDL or were left untreated for the corresponding
period, (each group with a minimum of n=10). (A) For hepatic immunohistochemistry
for -SMA,
representative sections are shown, Sham group: -SMA
-positive cells in the structure of blood vessels of portal area; Ro
5: numerous -SMA
positive cells around proliferating bile ducts. Immunoperoxidase technique
counterstained with Gill 2 hematoxylin Bar=100 µm. (B) Representative
Western blots ( -SMA
and endogenous control GAPDH) and quantifications are shown (means ±S.D.,
the sham-group was set to 100%), p<0.001 vs. BDL. |
TGF-ß1 expression was mildly increased in the groups that underwent BDL compared
to the Sham group. Ro in either dose didn’t decrease the TGF-ß1 expression (
Fig.
7).
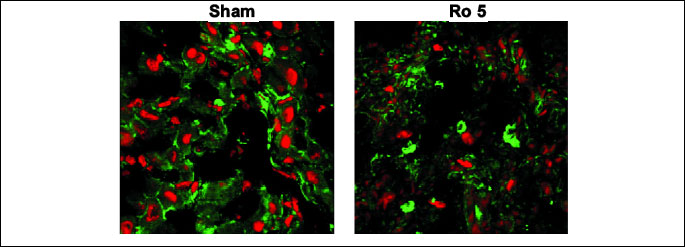 |
Fig.
7. The effects of the 6 days treatment with rosuvastatin (Ro5: 5 mg/bw
rosuvastatin orogastric) after bile duct ligation (BDL) in Wistar rats
on the TGF-ß1 expression by the liver parenchyma. Green channel
Rhodamine. Red channel DRAQ 5. Plan-Apochromat 63x oil objective. |
Ductular and hepatocyte proliferation were evaluated using the count of the number of newly formed bile ducts and the PCNA expression in the parenchyma and in the bile duct epithelium.
When assessing the number of newly formed bile ducts (
Table 3), rats
from BDL group showed typical ductular reaction characterized by marked increase
in the number of biliary epithelial cells. In the Ro 10 group, proliferation
of biliary epithelial cells was observed. The number of bile ducts was significantly
increased in all the groups as compared to the sham operated one (p<0.05). The
administration of Ro further increased the number of ducts as compared to the
group that underwent bile duct ligation, but this augmentation was not statistically
significant as compared to BDL group.
By immunohistochemistry, the number of PCNA positive cells in the bile duct
epithelium was significantly increased in the BDL, Ro 5 and Ro 10 groups as
compared to the Sham operated one (
Fig. 8A, 8B). When the number of PCNA
positive cells was calculated in the rest of the hepatic parenchyma (
Fig.
9A, 9B), we found that BDL increased significantly the number of PCNA positive
cells, as did the administration of 10 mg/bw rosuvastatin. This group (Ro 10)
had also a significantly higher number of PCNA positive cells as compared to
the one that was subjected only to bile duct ligation.
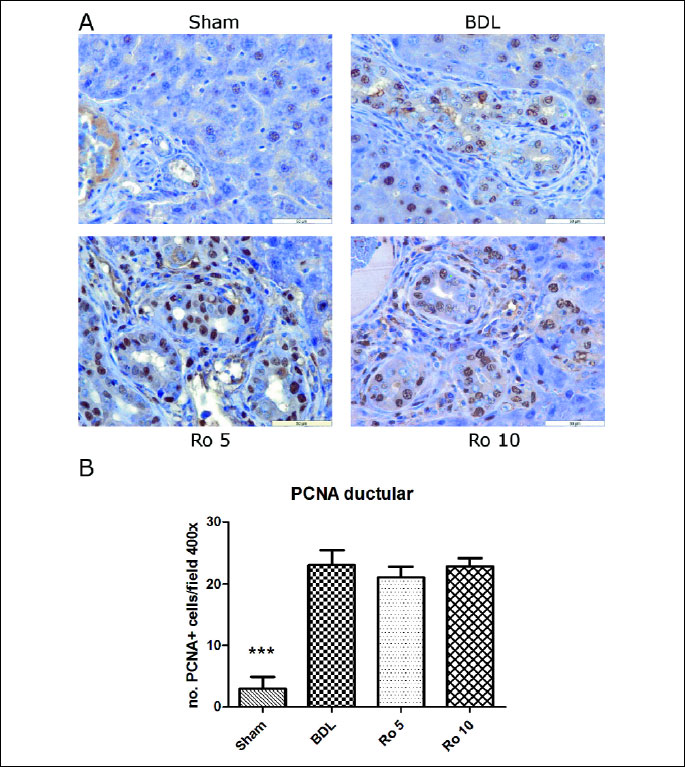 |
Fig.
8. PCNA expression by proliferating cholangiocytes in the liver of
Wistar rats that were treated 6 days with rosuvastatin (Ro 5: 5 mg/bw
rosuvastatin orogastric; Ro 10: 10 mg/bw rosuvastatin orogastric) after
bile duct ligation (BDL). (A) Immunoperoxidase technique counterstaind
with Gill 2 hematoxylin. Original magnification of x400. Scale bar=50
µm. (B) The number of PCNA positive cells was assessed by
counting 500 cells in non-overlapping fields for each slide and the data
expressed as percentage of positive cells. The data were analyzed by one-way
ANOVA followed by the Tukey´s multiple comparisons posttest using
GraphPad Prism 5.0 software (GraphPad, San Diego, SUA). The data were
expressed as means ±S.D.; *** p<0.001 as compared to the BDL
group. |
 |
Fig.
9. PCNA expression in the hepatic parenchyma of Wistar rats that were
treated 6 days with rosuvastatin (Ro 5: 5 mg/bw rosuvastatin orogastric;
Ro 10: 10 mg/bw rosuvastatin orogastric) after bile duct ligation (BDL).
(A) Immunoperoxidase technique counterstaind with Gill 2 hematoxylin.
Original magnification of x400. Scale bar=50 µm. (B) The
number of PCNA positive cells was assessed by counting 500 cells in non-overlapping
fields for each slide and the data expressed as percentage of positive
cells. The data were analyzed by one-way ANOVA followed by the Tukey´s
multiple comparisons posttest using GraphPad Prism 5.0 software (GraphPad,
San Diego, SUA). The data were expressed as means ±S.D.; *p<0.05
vs. BDL. |
DISCUSSION
Up to date, both the retrospective clinical studies and those carried out on animal models that studied the administration of statins in cholestasis weren’t able to offer definitive answers regarding the beneficial or noxious effect of this association. It seems, none the less, that the benefits far outweigh the risks for the patients with both dyslipidemia and cholestasis and so the administration of statins in this situation may not be a major concern (20). The experimental models, most of them on extrahepatic cholestasis, raise questions primarily because they are directed towards the initial phases of cholestasis after bile duct ligation and secondly due to the fact that they can offer valuable information on some other mechanisms involved in liver injury, such as inflammation, oxidative stress and fibrogenesis. Also, the bile duct ligation model can be valuable to study other associated pathological conditions, not only the liver injury, for example intestinal bleeding (21), or acute pancreatitis (22). As such, the clinical and experimental information that emerges from the studies is not perfectly superimposable (20). Recent experiments carried out on rats (23) showed that the administration of statins in the initial stages of BDL-induced cholestasis, alter the normal adaptive responses and tend to counteract the hepatic injury produced by cholestasis, and they are coordinated by nuclear receptors, mainly the farnesoid X receptor (FXR). This might be an explanation for the defavorable effects of statins in experimental cholestasis. Not even these results offer a definitive answer, because these changes were found at the level of mRNA, they were not proven at the protein expression level, and their relevance to human subjects remains to be established.
In our study, we aimed to explore the
in vivo effects of early rosuvastatin
administration, in different doses, on BDL-induced cholestasis. We targeted
the mechanisms highlighted by previous studies to be involved in the liver injury
associated to this pathological condition: oxidative stress, inflammation, cholangiocytes
and hepatocytes proliferation and fibrosis. Our study was centered on the liver,
but we also assessed the oxidative stress in plasma, brain and kidney. When
we designed this study we had taken into consideration the research carried
out by Dirlik
et al., which evaluated the ultrastructural and oxidative
stress changes found after BDL. The authors had concluded that the first 5 days
after acute BDL are the most critical and that cholestatic liver injury was
established and became obvious on the 5
th day
after acute BDL. Thus, they recommend testing the therapeutic effect of pharmaceutical
agents after the 5
th day from the BDL (24).
Our results have highlighted different types of effects that might be classified as “good” and “bad”, depending on the assessed mechanism. Normally, experimental BDL induces a bile duct-directed inflammatory response that leads to bile duct injury associated with biliary proliferation. Proliferating cholangiocytes influence other cell types: vascular endothelial cells, portal fibroblasts, hepatic stellate cells (HSC) (25) and these interactions will further determine the rapid occurrence of significant liver fibrosis (26).
As an overall look, we didn’t find an increase in mortality after BDL, nor after the simultaneous administration of Ro, but we found a slight reduction effect on body weight and an increase of liver weight with a similar tendency, both for BDL and BDL+Ro. As found by other authors (27, 28), in our study BDL produced oxidative stress in the liver, as shown by a significant increased level of MDA, PC, and decreased GSH/GSSG ratio. In addition, we did not obtain oxidative stress in plasma, kidney and brain. AST and ALT plasma levels, detected 7 days after the BDL, were increased, as well as GGT and bilirubin levels. Our results are in concordance with other studies (4, 9) that found increased levels of these parameters 7 days from BDL. Histological examination performed in our study showed significantly higher scores of necrosis and inflammation in the BDL group, with no obvious fibrosis, as compared to controls and also a ductular reaction with an increased number of newly formed bile ducts. In accordance to these findings, we obtained an increased expression of TNFR2 receptor, the activation of Kupffer cells, with no induction or activation of NF-

B. Normally, NF-

B responds directly to oxidative stress (29). Lipid peroxides and GSH depletion cause the phosphorylation and the subsequent degradation of the inhibitor of NF-

B, a critical step for NF-

B activation.
We have also confirmed increased levels of TGF-ß1 in the group that underwent BDL. These are anticipated findings, in accordance to the existing understanding concerning cholestatic liver disease.
In the meantime, a lot of factors, not only cytokines and chemokines (TGF-ß1,
TNF-

, IL-6, IL-8,
NO,
etc.) from ductal epithelium, but also oxidative stress, can activate
the HSC. Among them, TGF-ß1, derived from both paracrine and autocrine sources,
is the most potent fibrogenic cytokine in the liver (30). Once activated, TGF-ß1
leads to induction of collagen production. Quiescent HSC are induced by TGF-ß1
to transdifferentiate into myofibroblasts which express

-SMA
(31). Both the semiquantitative score of the

-SMA
positive cells and the quantitative evaluation by western blot of

-SMA
revealed a moderate increase of this protein in the liver of the rats that underwent
bile duct ligation. In the meantime, cellular proliferation is a compensatory
pathological reaction to hepatic injury, which can be evaluated by the detection
of cell mitosis or proliferation related markers (32). The level of expression
of PCNA, a molecular marker highly associated with cell cycle and proliferation
(33), was found to be significantly increased in mice and rats with BDL (1).
In our study, we have also found that, after BDL, the number of PCNA positive
cells was markedly increased both in the liver parenchyma and in the bile ducts.
The administration of Ro, in both doses, didn’t influence the mortality, nor the body weight of the animals or the weight of the liver. Our results showed that both doses of Ro determined an increased hepato-cytolysis evaluated through AST and ALT plasma levels, as compared both to BDL and Sham group, while cholestasis indices (GGT and BT) were improved. Also, Ro, daily administered, in either dose, maintained the oxidative stress alterations produced by BDL in the liver, with significant increase of MDA and PC and decreased GSH/GSSG ratio. In our study the early administration of Ro to the rats with BDL did not modify the expression of the profibrotic cytokine TGF-ß1 and exacerbated the inflammation, in comparison with the BDL group, evaluated by the necro-inflammatory scores and TNFR2 expression. Ro administration increased the number of ducts as compared to the group that underwent bile duct ligation, and the number of PCNA positive cells in the ducts and parenchyma. So, we didn’t find any beneficial effects of Ro administration on oxidative stress, inflammation, cholangiocytes proliferation, or expression of the profibrotic cytokine TGF-ß1. Other studies have pointed similar findings, with an increase in the level of oxidative stress associated with liver injury after statins administration (5), an increase in the levels of AST and ALT in day 7 of statins’ administration after BDL: atorvastatin (9) and fluvastatin (5). However, the cholestasis indices were improved, as shown also for Ro by Awad and Kamel (11). The increased level of AST, ALT in our experiment could be considered as an indirect sign of inflammation, as stated also by others (9).

-SMA didn’t seem
to be decreased by either dose of statin, when assessed through a semi-quantitative
method. But, at the quantitative evaluation, we clearly demonstrated that Ro
10 decreased a-SMA. The data are in agreement with the study of Trebicka
et
al. that showed similar findings after the administration of atorvastatin
(15 mg/bw) for 7 days after bile duct ligation (9), probably by the effect exerted
on the activation of HSC. That is a beneficial effect of Ro administration on
early stages of fibrosis installation.
The most unexpected result of our study was the effect of Ro on NF-

B
activation. Oxidative stress and TNF-

are known as NF-

B
activators. Both BDL and combined BDL+Ro administration determined oxidative
stress and inflammation in the liver. However, even though NF-

B
was nor induced, nor activated during BDL condition, the concomitant treatment
with Ro inhibited both the induction and the activation of NF-

B.
There are studies showing that inhibitors of NF-

B
activity exert a therapeutic effect on cholestatic liver injury in rats with
BDL through anti-inflammatory and antioxidant actions (34). It is possible that
Ro inhibits the NF-

B
induction/activation through a direct mechanism, which could not be validated
at this stage of our research.
When comparing the results on different mechanisms of the 2 doses of Ro, we
didn’t find a clear dose dependent effect. However, Ro 10, in comparison to
Ro 5, decreased more effectively GGT level and

-SMA,
decreased the Kupffer cells activation, but determined a higher necro-inflammatory
score, an increased expression of TNFR2, and the generation of a greater number
of PCNA positive cells in epithelial ducts. There were no significant differences
regarding NF-

B
induction and activation. It seems that higher doses of Ro during early stages
of cholestasis could have better effects on fibrosis onset and cholestasis,
but potentate inflammation and cholagiocytes’proliferation.
Our results cannot give definitive answers, since there are studies stating that at 7 and, respectively, 10 days after bile duct ligation the levels of MDA are lowered and the antioxidant defenses represented by SOD, CAT and GSH are increased (11). Decreased AST and ALT levels as compared to BDL group were also obtained for fluvastatin (35) and simvastatin (6). The differences between the results of our study and the studies sustaining the antioxidant and anti-inflammatory effects of statins could be explained by the existing differences between the experimental design in each case, regarding the type of animal, gender, the use of particular doses and type of statins, the moment of onset for the statins’ administration, the duration of treatment, the moment of evaluation. Progesterone, both in male and female, and follicle-stimulating hormone (FSH) stimulate proliferation of cholangiocytes (36) raising the question if gender cannot explain the differences between experimental models using male or female rats with statins and BDL.
For example, a part of our experiment seems to be similar to that performed by Awad and Kamel (11): 10 mg/bw of Ro administered daily, 7 days, after BDL. But on a closer look, the experiment is performed on male Sprague-Dawley rats, the administration of Ro begun from the third day after BDL, and the moment of evaluation was ten days after surgery. In our experiment we used female Wistar rats, Ro was administered from the first day after BDL, and the evaluation was seven days after surgery.
In conclusion, the administration of Ro in the early stages of cholestasis in
our study proved to have a beneficial effect by decreasing

-SMA,
depending on the administered dose. In the meantime, Ro didn’t exert systemic
oxidative stress effects and decreased the parameters of cholestasis. However,
Ro increased hepato-cytolysis, oxidative stress and inflammation in the liver,
maintained the increased levels of pro-fibrotic cytokineTGF-ß1 as well as the
number of proliferating cells in the ducts. As a particular finding, we notice
that Ro inhibits the induction and the activation of NF-

B,
which could be a beneficial effect. Further studies must be carried out in order
to clearly establish the ratio between risks and benefits for Ro administration
in early stages of cholestasis.
Acknowledgements:
This work was supported by the Ministry of Education, Research and Youth by
PN II Program (12-131/2008). We gratefully thank Mr Remus Moldovan for animal
handling. We also thank to Doina Daicoviciu and Nicoleta Decea for their help
regarding oxidative stress parameters’ assessment. We also thank Mr Alupei Marius
and Dr Manuela Banciu for their contribution to western blot assessment.
Conflict of interests: None declared.
REFERENCES
- Tomur A, Kanter M, Gurel A, Erboga M. The efficiency of CAPE on retardation of hepatic fibrosis in biliary obstructed rats. J Mol Histol 2011; 42: 451-458.
- Anan A, Baskin-Bey ES, Isomoto H. Proteasome inhibition attenuates hepatic injury in the bile duct-ligated mouse. Am J Physiol Gastrointest Liver Physiol 2006; 291: G709-G716.
- Forbes SJ, Parola M. Liver fibrogenic cells. Best Pract Res Clin Gastroenterol 2011; 25: 207-217.
- Olteanu D, Filip A, Muresan A, et al. The effects of chitosan and low dose dexamethasone on extrahepatic cholestasis after bile duct ligation in Wistar rats Acta Physiol Hung 2012; 99: 61-73.
- Lotkova H, Stankova P, Rousar T, et al. Deteriorating effect of fluvastatin on the cholestatic liver injury induced by bile duct ligation in rats. Gen Physiol Biophys 2011; 30: 66-74.
- Dold S, Laschke MW, Lavasani S, Menger MD, Jeppsson, Thorlacius H. Simvastatin protects against cholestasis-induced liver injury. Br J Pharmacol 2009; 156: 466-474.
- Gianella A, Nobili E, Abbate M, et al. Rosuvastatin treatment prevents progressive kidney inflammation and fibrosis in stroke-prone rats. Am J Pathol 2007; 170: 1165-1177.
- Oberti F, Pilette C, Rifflet H, et al. Effects of simvastatin, pentoxifylline and spironolactone on hepatic fibrosis and portal hypertension in rats with bile duct ligation. J Hepatol 1997; 26: 1363-1371.
- Trebicka J, Hennenberg M, Odenthal M, et al. Atorvastatin attenuates hepatic fibrosis in rats after bile duct ligation via decreased turnover of hepatic stellate cells. J Hepatol 2010; 53: 702-712.
- Martin PD, Warwick MJ, Dane AL, et al. Metabolism, excretion, and pharmacokinetics of rosuvastatin in healthy volunteers. Clin Ther 2003; 25: 2822-2835.
- Awad AS, Kamel R. Effect of rosuvastatin on cholestasis-induced hepatic injury in rat. Livers J Biochem Mol Toxicol 2010; 24: 89-94.
- Bradford MM. A rapid and sensitive method for the quantitation of microgram quantities of protein utilizing the principle of protein-dye binding. Anal Biochem 1976; 72: 248-254.
- Conti M, Moran PC, Levillain P. Improved fluorimetric determination of malondialdehyde. Clin Chem 1991; 37: 1273-1275.
- Reznick AZ, Packer L. Oxidative damage to proteins spectrophotometric method for carbonyl assay. Methods Enzymol 1994; 233: 357-363.
- Hu ML. Measurement of protein thiol groups and glutathion in plasma. Methods Enzymol 1994; 233: 380-384.
- Floreani M, Petrone M, Debetto P, Palatini P. A comparison between different methods for the determination of reduced and oxidized glutathione in mammalian tissues. Free Radic Res 1997; 26: 449-455.
- Bergmeyer HU, Scheibe P, Wahlefeld AW. Optimization of methods for aspartate aminotransferase and alanine aminotransferase. Clin Chem 1978; 24: 58-73.
- Knodell RG, Ishak KG, Black WC, et al. Formulation and application of a numerical scoring system for assessing histological activity in asymptomatic chronic active hepatitis. Hepatology 1981; 1: 431-435.
- Tabaran F, Catoi C, Clichici S, et al. Immunohistochemical quantification of the tumor necrosis factor (TNF) receptor II expression in the hepatic tissue after systemic administration of the DNA-SWCNT. Bull USAMV 2011; 68: 370-375.
- Kuver R. Effects of statins on cholestasis: good, bad or indifferent? J Gastroenterol Hepatol 2011; 26: 1544-1551.
- Lichtenberger LM, Phan T, Okabe S. Aspirin’s ability to induce intestinal injury in rats is dependent on bile and can be reversed if pre-associated with phosphatidylcholine. J Physiol Pharmacol 2011; 62: 491-496.
- Petrovic I, Dobric I, Drmic D, et al. BPC 157 therapy to detriment sphincters failure-esophagitis-pancreatitis in rat and acute pancreatitis patients low sphincters pressure. J Physiol Pharmacol 2011; 62: 527-534.
- Kolouchova G, Brcakova E, Hirsova P, et al. Pravastatin modulates liver bile acid and cholesterol homeostasis in rats with chronic cholestasis. J Gastroenterol. Hepatol 2011; 26: 1544-1551.
- Dirlik M, Canbaz H, Dusmez A, et al. The monitoring of progress in apoptosis of liver cells in bile duct-ligated rats. Turk J Gastroenterol 2009; 20: 247-256.
- Yang F, Gaudio E, Onori P, Wise P, Alpini G, Glaser S. Mechanisms of biliary damage. J Cell Death 2010; 3: 13-21.
- Strazzabosco M, Fabris L, Spirli C. Pathophysiology of cholangiopathies. J Clin Gastroenterol 2005; 39(4 Suppl 2): S90-S102.
- Lu SC. Regulation of glutathione synthesis. Mol Aspects Med 2009; 30: 42-59.
- Serviddio G, Pereda J, Pallardo FV, et al. Ursodeoxycholic acid protects against secondary biliary cirrhosis in rats by preventing mitochondrial oxidative stress. Hepatology 2004; 39: 711-720.
- Schreck R, Ribor P, Baeuerle PA. Reactive oxygen intermediates as apparently widely used messengers in the activation of the NF-kappa B transcription factor and HIV-1. EMBO J 1991; 10: 2247-2258.
- Lee UE, Friedman SL. Mechanisms of hepatic fibrogenesis. Best Pract Res Clin Gastroenterol 2011; 25: 195-206.
- Kisseleva T, Brenner DA. Anti-fibrogenic strategies and the regression of fibrosis. Best Pract Res Clin Gastroenterol 2011; 25: 305-317.
- Colozza M, Azambuja E, Cardoso F, Sotiriou C, Larsimont D, Piccart MJ. Proliferative markers as prognostic and predictive tools in early breast cancer: where are we now? Ann Oncol 2005; 16: 1723-1739.
- Bhattacharyya NK, Chatterjee U, Sarkar S, Kundu AK. A study of proliferative activity, angiogenesis and nuclear grading in renal cell carcinoma. Indian J Pathol Microbiol 2008; 511:17-21.
- Demirbilek S, Akin M, Gurunluoglu K, et al. The NF-kappaB inhibitors attenuate hepatic injury in bile duct ligated rats. Pediatr Surg Int 2006; 22: 655-663.
- Demirbilek S, Tas E, Gurunluoglu K, et al. Fluvastatin reduced liver injury in rat model of extrahepatic cholestasis. Pediatr Surg Int 2007; 23: 155-162.
- Glaser SS, Onori P, Wise C, et al. Recent advances in the regulation of cholangiocyte proliferation and function during extrahepatic cholestasis. Dig Liver Dis 2010; 42: 245-252.