HYPERVENTILATION ASSISTS PROARRHYTHMIA DEVELOPMENT DURING DELAYED REPOLARIZATION IN CLOFILIUM-TREATED, ANAESTHETIZED, MECHANICALLY VENTILATED RABBITS
INTRODUCTION
It is known that serum potassium (K+) concentration is influenced by the arterial CO2 level. The partial pressure of CO2 (PCO2) is reduced by hyperventilation in the blood, which leads to respiratory alkalosis, and a consequent compensatory mechanism reduces serum K+ concentration (1, 2). It is also known that occurrence of arrhythmias is affected by the serum K+ level. In hypokalaemic conditions, greater in number and more serious arrhythmias develop during ischaemia and in acute myocardial infarction (3, 4). Also, hypokalaemia is one of the well-known risk factors of the severe proarrhythmic event, the life-threatening torsades de pointes ventricular arrhythmia (TdP) evoked by repolarization delaying drugs (5). It implies that hyperventilation and the resultant hypocapnia and hypokalaemia may facilitate the development of drug-induced TdP. However, the effect of hyperventilation on arrhythmia development during delayed repolarization has not been investigated yet.
Anaesthetized, ventilated rabbits are utilized in one of the most frequently used in vivo proarrhythmia models, and the rabbits are sensitized to the development of drug-induced TdP by intravenous administration of α1-adrenoceptor agonists (6, 7). This experimental model was developed by Carlsson et al., more than 25 years ago (6), and it has successfully been used for scientific investigations by several research groups. However, no data are available about the effect of mechanical ventilation induced respiratory alkalosis and hypocapnia on the occurrence of drug-induced arrhythmias in in vivo experimental proarrhythmia investigations. Thus, our aim was to examine the relationship between the blood gas values (especially PCO2) and development of arrhythmias during delayed repolarization in clofilium-treated, α1-adrenoceptor-stimulated, anaesthetized, mechanically ventilated rabbits. If there was a correlation between hypocapnia and occurrence of proarrhythmic events during drug-induced repolarization delay, then it would have clinical relevance, since mechanical ventilation can lead to respiratory alkalosis during surgeries and intensive therapy (2), meanwhile patients may be treated with various proarrhythmic cardiac and non-cardiac drugs that delay ventricular repolarization (8, 9).
MATERIALS AND METHODS
In the present study, a retrospective analysis of the blood gas and arrhythmia data of previous experimental investigations (10-12) was performed. The used experimental methods have already been published in detail (10-13), thus methods are only briefly described here.
Animals, general experimental method
Experiments were performed on male, New-Zealand White rabbits. The animal handling procedures were in accordance with the Guidance on the Operation of the Animals (Scientific Procedures) Act 1986, London, UK and also adhered to European Community Guidelines for the use of experimental animals. The experiments were conducted under the authority of Project License no. 40/1702 and approved by the University of Liverpool Animal Welfare Committee.
Animals were anaesthetized with Na-pentobarbitone (30 mg kg–1 i. v.). Trachea was cannulated and median sternotomy was performed. The open-chest rabbits were mechanically ventilated (Bioscience pump) with room air at a rate of 38 stroke min–1 and a stroke volume of ~6 ml kg–1 body weight and a positive end expiratory pressure of 1 – 2 cm H2O. The applied respiratory rate and stroke volume are in the physiological ranges of these parameters in rabbits (14). Leads I, II and III of the electrocardiogram (ECG) were recorded simultaneously, together with the monophasic action potential signal, at a sampling rate of 1000 Hz by the Po-Ne-Mah data acquisition system (Linton, Diss, Norfolk, USA). Blood gas values, serum pH and K+ values were measured with a Ciba Corning 850 pH/blood gas analyzer (Bayer, Neuburg, Berks, UK) in blood samples obtained from the femoral artery after the surgical preparation, in the last minute of the 20-min-long stabilization period, immediately before the start of the experimental protocol.
Experimental protocol
In the present study, the data of 29 animals were evaluated. Every animal received the same drug treatment, which is the following: phenylephrine (an α1-adrenoceptor agonist) and clofilium (a repolarization delaying agent inhibiting the rapid component of the delayed rectifier potassium current, IKr) were infused intravenously in increasing doses. Protocol consisted of three dosing cycles. In each cycle, phenylephrine infusion was the same: 75 nmol kg–1 min–1 for 15 minutes, 150 nmol kg–1 min–1 for 3 minutes, 225 nmol kg–1 min–1 for 3 minutes, 300 nmol kg–1 min–1 for 3 minutes. At the fifth minute of the first cycle, a simultaneous clofilium infusion (25 nmol kg–1 min–1) was started and lasted for 19 minutes. Both drug infusions were paused at the end of the cycle and were followed by a 10-min drug-free interval. Dose of phenylephrine did not change in the second and third cycle, whereas dose of clofilium was increased to 60 nmol kg–1 min–1 in the second cycle and 200 nmol kg–1 min–1 in the third cycle (Fig. 1).
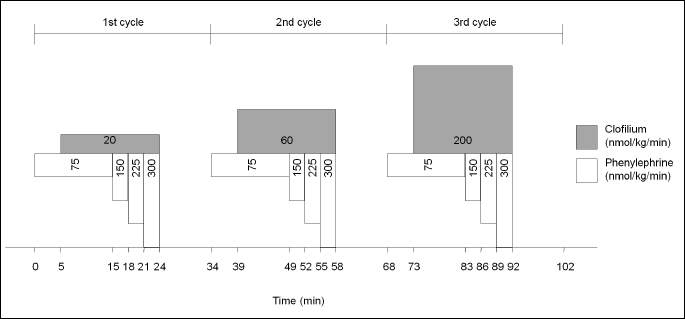
In the present study, the 29 animals were retrospectively divided into two groups according to the presence or absence of clofilium-induced TdP, i.e. animals that experienced clofilium-induced TdP were allocated into the TdP+ group (n = 15 animals), and animals that did not experience clofilium-induced TdP were allocated into the TdP– group (n = 14 animals).
ECG analysis and arrhythmia diagnosis
The evaluated experiments served as ‘positive controls’ in earlier pharmacological investigations (10-12). The experiments were performed in a randomized manner, and the ECG analysis was performed in a randomized and blinded manner.
ECG intervals were measured manually in stable sinus rhythm, as described previously (15, 16). QT intervals were measured from the beginning of the Q wave to the end of the T wave or U wave (if present). The values for QT interval were corrected for heart rate using the equation: QTc = QT-0.704(RR-250), which has been shown previously to be appropriate for these pentobarbitone-anaesthetized rabbits (17). The heart rate, the QT interval, and the rate corrected QT interval (QTc) were determined at baseline (immediately before the start of the protocol), 10 min after the start of first clofilium infusion, and before TdP development in the TdP+ group, or at equivalent time point in the TdP– group.
The incidence, the time to onset and the duration of ventricular arrhythmias were obtained. Ventricular premature beats, bigeminy, salvos and ventricular fibrillation were defined according to the Lambeth Conventions (18). TdP was defined as a polymorphic ventricular tachycardia where clear twisting of the QRS complexes around the isoelectric axis could be seen in at least one ECG lead (10-12). Runs of four or more ventricular premature beats without the torsades-like twisting QRS morphology were differentiated from TdP and were defined as ventricular tachycardia.
Drugs
Clofilium (clofilium tosylate) and phenylephrine (L-phenylephrine HCl) were purchased from Sigma Chemical Co. (Poole, Dorset, UK); sodium pentobarbitone (Sagatal®) was bought from National Veterinary Supplies Ltd (Stoke-on-Trent, UK). Clofilium and phenylephrine was dissolved in saline. For further details see references (10-12).
Statistical analysis
Continuous data are shown as mean ± standard error of the mean (S.E.M.). Arrhythmia onset times, and baseline pH, PCO2 and K+ values were compared with independent samples T-test between the TdP+ and TdP– groups. The heart rate, QT and QTc values were compared with repeated measures analysis of variance between the TdP+ and TdP– groups. Correlation between PCO2 and serum K+ level as well as correlation between PCO2, serum K+ level and time to onset of arrhythmias were examined with linear regression in all the 29 animals (irrespective of TdP+ and TdP– groups). The correlations i) between serum PCO2 and serum K+ level, ii) between PCO2 and arrhythmia onset time, and iii) between serum potassium level and arrhythmia onset time were examined with analysis of covariance in the TdP+ and TdP– groups (TdP+ versus TdP– group, as the categorical grouping variable). Differences were considered statistically significant when P < 0.05.
RESULTS
The rate corrected QT interval
As expected, the repolarization delaying clofilium significantly prolonged the QTc interval in the TdP+ and TdP– groups during the experiments; there was no significant difference in the QTc interval between the TdP+ and TdP– groups at the time points of the measurement (TdP+ group: 195 ± 4 ms, 216 ± 6 ms and 227 ± 8 ms at baseline, 10 min after the start of the clofilium infusion, and before TdP development, respectively; TdP– group: 200 ± 4 ms, 214 ± 4 ms and 222 ± 4 ms at baseline, 10 min after the start of the clofilium infusion, and at equivalent time point to the TdP development in the TdP+ group, respectively).
Baseline PCO2 and K+ values, time to onset of the first arrhythmia, other blood gas values
Mechanical ventilation induced respiratory alkalosis in the animals. There was no significant difference in the baseline pH, PCO2 and K+ values between the TdP+ and TdP– groups (Table 1). It implies that there is no relationship between development of clofilium-induced TdP and baseline pH, PCO2 and K+ values. There was no significant difference in baseline serum Na+, Cl–, HCO3–, base excess and PO2 values between the TdP+ and TdP– groups, too; values were in the normal range (data not shown).

As we described earlier, TdP was preceded by less complex arrhythmias (Fig. 2) (7, 19, 20), which frequently developed in the TdP– group, too. The first arrhythmia developed earlier in the TdP+ group than in TdP– group (Table 1). TdP occurred in the TdP+ group at an average of 2479 ± 261 s after the start of the protocol.
![]() |
Fig. 2. An example of clofilium-induced torsades de pointes type ventricular arrhythmia (TdP) with preceding non-TdP arrhythmias in anaesthetized rabbits. ECG I-III, Lead I, Lead II and Lead III limb lead electrocardiograms; BP arterial blood pressure; Epi MAP, epicardial monophasic action potential; VPB, ventricular premature beat; Sal2, a salvo consisting of 2 VPBs; Sal3, a salvo consisting of 3 VPBs. |
Examination of the relationship between the serum K+ and PCO2 values
A strong, positive correlation was found between the baseline serum K+ and PCO2 values of the animals, when the pooled data of the TdP+ and TdP– groups (n = 29) were analyzed (Fig. 3A).
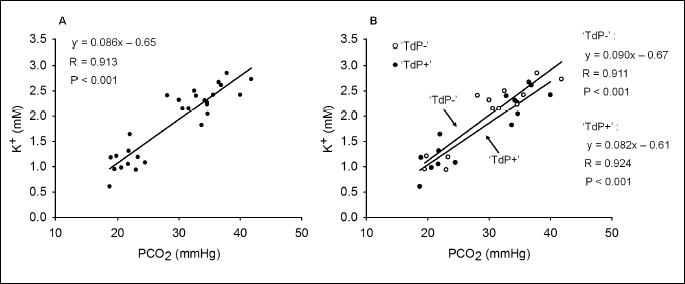
According to one of our hypotheses, the correlation between the PCO2 and K+ may be different in the two groups. Thus, the correlation between the PCO2 and serum K+ level was examined with analysis of covariance in the TdP+ and TdP– groups. Results show that the correlation between the baseline PCO2 and serum K+ values is remarkably close in both of the groups (Fig. 3B). Results of the analysis of covariance revealed that the positive linear correlation between the K+ and PCO2 values does not differ significantly in the TdP+ and TdP– groups. This suggests that TdP development was not determined by any difference in the correlation between PCO2 and K+ values in the two groups.
Examination of the relationship between the serum K+ and time to onset of the first arrhythmia
Although data were scattering, a significant, positive, linear correlation was found between the baseline serum K+ value and time to onset of the first arrhythmia, when the pooled data of the TdP+ and TdP– groups (n = 29) were analyzed with linear regression (Fig. 4A). Thus, the lower the baseline serum K+ value is, the earlier the first drug-induced arrhythmia develops in the anaesthetized rabbit model of TdP.
The relationship between the serum K+ and time to onset of the first arrhythmia was examined in the TdP+ and TdP– groups, too. In the TdP+ group, the correlation between serum K+ and time to onset of the first arrhythmia was significant, but the correlation coefficient was low. In the TdP– group, data are very scattering, thus the correlation between serum K+ and time to onset of the first arrhythmia was found not to be significant (Fig. 4B).
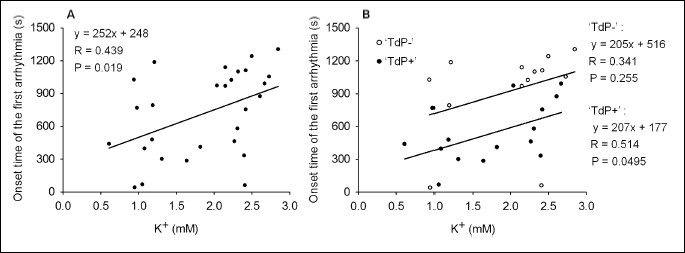
Examination of the relationship between the PCO2 and time to onset of the first arrhythmia
The relationship between the baseline PCO2 and time to onset of the first arrhythmia was directly examined, too. Although data were scattering, a significant positive linear correlation was found between the baseline PCO2 and time to onset of the first arrhythmia, when the pooled data of the TdP+ and TdP– groups (n = 29) were analyzed with linear regression (Fig. 5A). This relationship is similar to the previously described positive linear correlation between the serum K+ and time to onset of the first arrhythmia. According to the present results, the lower the baseline PCO2 value is, the earlier the first arrhythmia develops in the anaesthetized rabbit model of TdP.
The relationship between the PCO2 and time to onset of the first arrhythmia was examined in the TdP+ and TdP– groups, too. A significant, positive, linear correlation was found between the baseline PCO2 values and time to onset of the first arrhythmia in both of the groups. Accordingly, the first arrhythmia develops earlier in case of hypocapnia (Fig. 5B). There was no significant difference in the slope of the regression line between the TdP+ and TdP– groups. However, a significant difference was found in the intercept of the regression line between the TdP+ and TdP– groups (analysis of covariance). Despite having similar PCO2 values in the TdP+ and TdP– groups, the first arrhythmia develops earlier in TdP+ group than in TdP– group. As no significant differences were found in the mean PCO2 and the mean serum K+ levels between the TdP+ and TdP– groups, it is concluded that some other, here not examined factors also contribute to arrhythmia development in the anaesthetized rabbit model of TdP.

DISCUSSION
The present study examined the effects of the PCO2 and serum K+ on the occurrence of arrhythmias during clofilium-induced repolarization delay in anaesthetized, mechanically ventilated, open-chest rabbits. A strong, positive, linear correlation was found between the PCO2 and serum K+ values, which means that acute hypocapnia results in acute hypokalaemia. Results show that occurrence of clofilium-induced arrhythmias is affected by the PCO2 and serum K+ values; hypocapnia and hypokalaemia assist early development of arrhythmias. Present data also show that first arrhythmias occurred earlier in the TdP+ group than in the TdP– group, though no difference was found either in the mean K+ concentration or in the PCO2 or in the correlation between these two parameters between the TdP+ and TdP– groups. Thus, in the rabbit model of TdP, occurrence of TdP and other arrhythmias also depend on other, here not examined factors, e.g. the activity of the autonomic nervous system (12, 21), inhomogeneity of the repolarization (20, 22), and the applied dose of the anaesthetic (pentobarbitone) (23).
Hyperventilation syndrome: acute hypocapnia results in acute hypokalaemia
In the present experiments, we found a remarkably close, positive, linear correlation between PCO2 and K+ values in anaesthetized, open-chest, mechanically ventilated rabbits. The noticed, low serum K+ values are clearly related to the process of anaesthesia and mechanical ventilation, since the measured K+ concentrations were normal in the alert, spontaneously breathing rabbits before the start of anaesthesia and mechanical ventilation (12). Acute respiratory alkalosis with consequent hypokalaemia frequently develop as side effects of mechanical ventilation in patients during surgeries (1, 2), and in patients treated in intensive care units (24). Hypokalaemia, as a consequence of respiratory alkalosis, has already been reported and examined in dogs (25), too. Hyperventilation evoked by mechanical ventilation rapidly and progressively induces serum electrolyte disturbances, e.g. hypokalaemia and hypomagnesemia (1, 2, 24), which markedly increase arrhythmia risk (26). Thus, avoiding respiratory alkalosis and strict monitoring of PCO2 and serum K+ levels are recommended in patients with mechanical ventilation (1, 2, 24).
Hyperventilation syndrome (hypocapnia with hypokalaemia and hypomagnesemia) can also be induced by spontaneous hyperventilation not related to mechanical ventilation in conditions like surgical stress in spinal anaesthesia (27), alcohol withdrawal (28) (29), and stroke in the brainstem (30).
Respiratory and metabolic alkalosis lead to hypokalaemia via the ‘cation shift’. Since extracellular H+ concentration decreases in alkalosis, a compensatory mechanism increases the exchange of H+ and K+ ions between the intracellular and extracellular space. As a result, H+ concentration increases and K+ concentration decreases in the extracellular space (26). The noticed close, positive correlation between PCO2 and serum K+ values suggests that the parameters of the mechanical ventilation should be controlled tightly in in vivo proarrhythmia experiments, as well as in clinical circumstances (1, 2, 24).
Hypokalaemia promotes development of serious ventricular arrhythmias, e.g. ventricular fibrillation and drug-induced torsades de pointes
It is well-documented, but probably not sufficiently emphasized in the everyday clinical practice, that hypokalaemia markedly increases the incidence of serious arrhythmias e.g. ventricular fibrillation in ischaemia and acute myocardial infarction (4). Severe arrhythmogenic effect of hypokalaemia was demonstrated in an earlier investigation showing that the incidence of ischemic ventricular fibrillation increased from 30% to 90%, when K+ concentration of the perfusion solution was decreased from 5 mmol/l to 3 mmol/l in isolated, Langendorff perfused rat hearts (31).
Hypokalaemia is arrhythmogenic in acquired long QT syndrome, too. The incidence of the drug-induced, life threatening TdP ventricular arrhythmia is markedly increased by low serum K+ concentrations (5). Clofilium, which was applied in the present experiments, prolongs repolarization and can be used only for experimental investigations because of its high proarrhythmic activity. However, many cardiac and non-cardiac drugs in clinical use delays repolarization and exert proarrhythmic liability (e.g. amiodarone, moxifloxacin, erythromycin, azythromycin, haloperidol, droperidol, methadone, etc.; for an up-to-date list of proarrhythmic drugs see https://crediblemeds.org). Interactions of frequently used medications e.g. ondansetron and famotidine together can also induce dangerous repolarization prolongation in patients (9). Repolarization delaying drugs may be applied frequently in mechanically ventilated patients, too; thus, it is very important to avoid contributing factors of TdP, e.g. hypokalaemia in these patients (32).
Hyperventilation and arrhythmias during mechanical ventilation
Until now, only few studies investigated the arrhythmogenic effect of hyperventilation directly. Studies with healthy adult and children volunteers showed that hyperventilation can induce repolarization disturbances e.g. prolongation of the QT interval, morphological changes of the ST segment and T wave in the ECG (33, 34); however no arrhythmias were reported in either of the investigations. On the other hand, when the risk factors of the occurring, haemodynamically compromising arrhythmias were examined in mechanically ventilated patients, one of the significant risk factors was found to be the pH greater than 7.42 (35). In accordance with this, our results imply that hypocapnia and respiratory alkalosis may increase the risk of development of arrhythmias during drug-induced repolarization delay, probably via the consequent hypokalaemia; however, further investigations are needed to verify this.
Conclusion
There is a close, positive, linear correlation between the PCO2 and serum K+ values in anaesthetized, mechanically ventilated, open-chest rabbits; hyperventilation causes acute hypocapnia with consequent acute hypokalaemia. According to our results, hypocapnia and hypokalaemia assist early development of arrhythmias during delayed repolarization in clofilium-treated rabbits. This implies that PCO2 and serum K+ should be controlled tightly during mechanical ventilation in experimental investigations and clinical settings when repolarization-delaying drugs are applied.
Acknowledgments: The late Dr. Susan J. Coker is thanked for supervising the experiments in her laboratory at the Department of Pharmacology and Therapeutics, The University of Liverpool, Liverpool, UK.
The experimental work was funded by the British Heart Foundation (PG96/100). This work was also supported by Hungarian Scientific Research Fund (OTKA PD 105882, OTKA NK-104331), National Development Agency and European Regional Fund (TAMOP-4.2.2-08/1-2008-0013, TAMOP-4.2.1/B-09/1/KONV-2010-0005, TAMOP-4.2.2/B-10/1-2010-0012, TAMOP-4.2.2.A-11/1/KONV-2012-0052, TAMOP-4.2.2.A-11/1/KONV-2012-0060, TAMOP-4.2.2.A-11/1/KONV-2012-0073), Hungary-Romania Cross-Border Cooperation Programme (HURO/1001/086/2.2.1_HURO-TWIN), and the UNKP-UNKP-16-4 New National Excellence Program of the Ministry of Human Capacities.
Dr. Attila S. Farkas was a holder of Janos Bolyai fellowship (BO/00794/12) of the Hungarian Academy of Sciences during this investigation.
Conflict of interests: None declared.
REFERENCES
- Sanchez MG, Finlayson DC. Dynamics of serum potassium change during acute respiratory alkalosis. Can Anaesth Soc J 1978; 25: 495-498.
- Edwards R, Winnie AP, Ramamurthy S. Acute hypocapneic hypokalemia: an latrogenic anesthetic complication. Anesth Analg 1977; 56: 786-792.
- Curtis MJ, Hearse DJ. Ischaemia-induced and reperfusion-induced arrhythmias differ in their sensitivity to potassium: implications for mechanisms of initiation and maintenance of ventricular fibrillation. J Mol Cell Cardiol 1989; 21: 21-40.
- Nordrehaug JE, von der Lippe G. Hypokalaemia and ventricular fibrillation in acute myocardial infarction. Br Heart J 1983; 50: 525-529.
- Farkas AS, Nattel S. Minimizing repolarization-related proarrhythmic risk in drug development and clinical practice. Drugs 2010; 70: 573-603.
- Carlsson L, Almgren O, Duker G. QTU-prolongation and torsades de pointes induced by putative class III antiarrhythmic agents in the rabbit: etiology and interventions. J Cardiovasc Pharmacol 1990; 16: 276-285.
- Farkas A, Lepran I, Papp JG. Comparison of the antiarrhythmic and the proarrhythmic effect of almokalant in anaesthetised rabbits. Eur J Pharmacol 1998; 346: 245-253.
- Alexandrou AJ, Milnes JT, Sun SZ, et al. The human ether-a’-go-go related gene (hERG) K+ channel blockade by the investigative selective-serotonin reuptake inhibitor CONA-437: limited dependence on S6 aromatic residues. J Physiol Pharmacol 2014; 65: 511-523.
- Armahizer MJ, Seybert AL, Smithburger PL, Kane-Gill SL. Drug-drug interactions contributing to QT prolongation in cardiac intensive care units. J Crit Care 2013; 28: 243-249.
- Farkas A, Coker SJ. Limited induction of torsade de pointes by terikalant and erythromycin in an in vivo model. Eur J Pharmacol 2002; 449: 143-153.
- Farkas A, Coker SJ. Prevention of clofilium-induced torsade de pointes by prostaglandin E2 does not involve ATP-dependent K+ channels. Eur J Pharmacol 2003; 472: 189-196.
- Farkas A, Dempster J, Coker SJ. Importance of vagally mediated bradycardia for the induction of torsade de pointes in an in vivo model. Br J Pharmacol 2008; 154: 958-970.
- Lengyel C, Varro A, Tabori K, Papp JG, Baczko I. Combined pharmacological block of I(Kr) and I(Ks) increases short-term QT interval variability and provokes torsades de pointes. Br J Pharmacol 2007; 151: 941-951.
- Leegaard F. The respiration and the respiratory gas exchange in experimental pneumonia. Acta Med Scand 1927; 76: 401-421.
- Farkas A, Batey AJ, Coker SJ. How to measure electrocardiographic QT interval in the anaesthetized rabbit. J Pharmacol Toxicol Methods 2004; 50: 175-185.
- Szepesi J, Acsai K, Sebok Z, et al. Comparison of the efficiency of Na+/Ca2+ exchanger or Na+/H+ exchanger inhibition and their combination in reducing coronary reperfusion-induced arrhythmias. J Physiol Pharmacol 2015; 66: 215-226.
- Batey AJ, Coker SJ. Proarrhythmic potential of halofantrine, terfenadine and clofilium in a modified in vivo model of torsade de pointes. Br J Pharmacol 2002; 135: 1003-1012.
- Walker MJ, Curtis MJ, Hearse DJ, et al. The Lambeth Conventions: guidelines for the study of arrhythmias in ischaemia infarction, and reperfusion. Cardiovasc Res 1988; 22: 447-455.
- Farkas A, Lepran I, Papp JG. Proarrhythmic effects of intravenous quinidine, amiodarone, D-sotalol, and almokalant in the anesthetized rabbit model of torsade de pointes. J Cardiovasc Pharmacol 2002; 39: 287-297.
- Farkas AS, Rudas L, Makra P, et al. Biomarkers and endogenous determinants of dofetilide-induced torsades de pointes in alpha(1) -adrenoceptor-stimulated, anaesthetized rabbits. Br J Pharmacol 2010; 161: 1477-1495.
- Farkas AS, Acsai K, Toth A, et al. Importance of extracardiac alphα1-adrenoceptor stimulation in assisting dofetilide to induce torsade de pointes in rabbit hearts. Eur J Pharmacol 2006; 537: 118-125.
- Yasuda C, Yasuda S, Yamashita H, Okada J, Hisada T, Sugiura S. The human ether-a-go-go-related gene (hERG) current inhibition selectively prolongs action potential of midmyocardial cells to augment transmural dispersion. J Physiol Pharmacol 2015; 66: 599-607
- Vincze D, Farkas AS, Rudas L, et al. Relevance of anaesthesia for dofetilide-induced torsades de pointes in alphα1-adrenoceptor-stimulated rabbits. Br J Pharmacol 2008; 153: 75-89.
- Watt JW, Silva P. Respiratory alkalosis and associated electrolytes in long-term ventilator dependent persons with tetraplegia. Spinal Cord 2001; 39: 557-563.
- Muir WW, Wagner AE, Buchanan C. Effects of acute hyperventilation on serum potassium in the dog. Vet Surg 1990; 19: 83-87.
- El-Sherif N, Turitto G. Electrolyte disorders and arrhythmogenesis. Cardiol J 2011; 18: 233-245.
- Moon HS, Lee SK, Chung JH, In CB. Hypocalcemia and hypokalemia due to hyperventilation syndrome in spinal anesthesia - a case report. Korean J Anesthesiol 2011; 61: 519-523.
- Elisaf M, Merkouropoulos M, Tsianos EV, Siamopoulos KC. Pathogenetic mechanisms of hypomagnesemia in alcoholic patients. J Trace Elem Med Biol 1995; 9: 210-214.
- Bebarova M, Matejovic P, Pasek M, Simurdova M, Simurda J. Dual effect of ethanol on inward rectifier potassium current IK1 in rat ventricular myocytes. J Physiol Pharmacol 2014; 65: 497-509.
- Ardhanari S, Gomathi SB, Anita L, Harish A, Ajit SM, Pandurangi UM. Brainstem infarct presenting as torsades de pointes. Indian Heart J 2009; 61: 368-370.
- Farkas A, Curtis MJ. Limited antifibrillatory effectiveness of clinically relevant concentrations of class I antiarrhythmics in isolated perfused rat hearts. J Cardiovasc Pharmacol 2002; 39: 412-424.
- Drew BJ, Ackerman MJ, Funk M, et al. Prevention of torsade de pointes in hospital settings: a scientific statement from the American Heart Association and the American College of Cardiology Foundation. Circulation 2010; 121: 1047-1060.
- Kannivelu A, Kudumula V, Bhole V. Effect of hyperventilation on rate corrected QT interval of children. Arch Dis Child 2013; 98: 103-106.
- Rutherford JJ, Clutton-Brock TH, Parkes MJ. Hypocapnia reduces the T wave of the electrocardiogram in normal human subjects. Am J Physiol Regul Integr Comp Physiol 2005; 289: R148-R155.
- Koh Y, Kim TH, Lim CM, et al. Risk factors for the development of hemodynamically significant cardiac arrhythmias in patients with mechanical ventilation. J Crit Care 2000; 15: 46-51.
A c c e p t e d : October 31, 2016