The pancreatic
secretory response to intestinal nutrients is mediated by a complex interplay
of neural and humoral mediators. The extrinsic parasympathetic (vagal) innervation
of the pancreas plays a major role in the control of pancreatic exocrine secretion.
Apart from the gastrointestinal tract, the pancreas is the only other peripheral
organ that has a significant intrinsic nerve plexus. After a short overview
over the extrinsic and intrinsic innervation of the pancreas, the present article
reports functionally findings regarding the cholinergic control of pancreatic
exocrine secretion and possible sites of interaction between cholinergic nerves
and other neural and hormonal mediators.
EXTRINSIC INNERVATION OF THE PANCREAS
Parasympathetic nerves
The parasympathetic nerve fibers innervating the
pancreas originate mainly from the dorsal vagal nucleus and partly from the
nucleus ambigous of the brain stem (1). Most of these fibers run within the
posterior vagal truncus (2) near the small curvature of the stomach and the
pylorus to the pancreas (3). Some of the fibers run intramurally along the pylorus
and the proximal part of the duodenum before joining the pancreas (4). The majority
of the vagal preganglionic fibers terminate on the pancreatic ganglia, performing
interneuronal synapses.
Sympathetic nerves
The pancreas receives its postganglionic sympathetic
innervation mainly from neurons with their cell bodies in the celiac and superior
mesenteric ganglia. These fibers are distributed to nerve cell bodies in the
pancreatic ganglia and to ducts, blood vessels, and islets. Some sympathetic
fibers run within the pancreatic parenchyma, directly terminating on acinar
cells. The sympathetic innervation of the exocrine pancreas, however, is modest
as compared with the islets and the pancreatic blood vessels (5).
INTRINSIC INNERVATION OF THE PANCREAS
Comparable to the enteric nevous system, an intrapancreatic
nervous system exists that enables a degree of independence of the pancreas
when cut off from the CNS and the gut. The morphological equivalent is an intrapancreatic
ganglionated plexus with terminal axons supplying both the endocrine and exocrine
portions of the organ (6, 7). The pancreatic ganglia are the nervous integration
centers of the pancreatic exocrine (and endocrine) secretion. They receive input
from vagal preganglionic, sympathetic postganglionic, sensory and enteric fibers.
Postganglionic nerve fibers surround almost every acinus, forming a periacinar
plexus containing cholinergic, noradrenergic, petidergic, and nitrergic fibers
which in majority terminate at the acinar cells (6—8). Only a subset of pancreatic
neurons appears to project to the islets (7).
CHOLINERGIC CONTROL OF PANCREATIC EXOCRINE
SECRETION
There is strong support for the hypothesis that
intrapancreatic postganglionic neurons regulate both enzyme and bicarbonate
secretion. These neurons are.activated by central input on the pancreatic ganglia
during the cephalic phase and by vagovagal reflexes initiated by gastric and
intestinal phase stimulation. Acetylcholine released by these neurons acts directly
on muscarinic receptors on acinar cells (and presumably duct cells) to elicit
secretion (5).
Role of the vagus nerves
Electrical stimulation of the vagal trunks in animals
(9, 10) and efferent vagal activation induced by insulin hypoglycemia in humans
(11) or by administration of the glucose antagonist 2-deoxyglucose in dogs (12)
have been shown to cause strong stimulation of pancreatic enzyme output. In
addition, the pancreatic enzyme response to intestinal perfusion with amino
acids, peptides and fatty acids, and to a meal is strongly inhibited by atropine
(13—17) and vagotomy (11, 14, 17, 18).
Experimental evidence for an enteropancreatic reflex has come from a study on
the latency of pancreatic enzyme response, i. e., the time required to measure
a significant increase in pancreatic enzyme output after a rapid intraduodenal
bolus application of tryptophan or sodium oleate compared with intraportal injection
of CCK (14). In this study performed in dogs, even the shortest possible circulation
time for a maximal dose of CCK (>30 s) was significantly longer than the observed
latency of amylase response to the intestinal nutrients (<20 s). Furthermore,
truncal vagotomy and atropine both increased the latency to the intestinal stimulants
10-fold but hat no effect on the latency to intraportal CCK. Thus it was concluded
that, at least in dogs, a cholinergic, vagovagal enteropancreatic reflex mediates
the early pancreatic enzyme response to intestinal stimulants. Studies on the
latency of the pancreatic fluid secretory response suggest that the early pancreatic
fluid responses to intestinal tryptophan or sodium oleate are also mediated,
at least in part,
via a vagovagal, cholinergic enteropancreatic reflex
(19).
Follow-up studies performing stepwise extrinsic denervation of the pancreas
ruled out possible splanchnic pathways of enteropancreatic reflexes (17). Celiac
and superior mesenteric ganglionectomy did not alter the pancreatic protein
reponse to intestinal tryptophan. Atropine significantly reduced the pancreatic
protein response to low loads of tryptophan before but not after truncal vagotomy.
These data suggest that, in dogs, the pancreatic protein response to intraduodenal
tryptophan is, at least in part, mediated by long, cholinergic enteropancreatic
reflexes with both afferent and efferent limb carried by the vagus nerves (17).
Histochemical examinations demonstrated that in the rat there are enteric neurons
that project to the pancreatic ganglia, thus providing anatomical evidence for
these enteropancreatic reflexes (20, 21).
Studies on the effect of truncal vagotomy or atropine on the pancreatic secretory
response to different loads of intestinal nutrients (15, 17) have shown.that
vagal cholinergic mechanisms are the major mediators of the pancreatic secretory
response to low loads of intestinal stimulants, whereas hormones mediate the
secretory response particularly to high loads of intestinal stimuli (18, 22).
Role of M1 receptors
Since at least three pharmacologically distinct
subtypes (M1, M2, M3) of the muscarinic receptor do exist, the question emerged,
which subtype is responsible for the vagal cholinergic control of pancreatic
exocrine secretion. Based upon studies in which the M3 receptors were pharmacologically
blocked, the M3 receptor was considered as the typical muscarinic receptor of
the acinar cell (23—26).
In-vivo studies in humans and dogs, however,
revealed a physiological role of M1 receptors in the control of pancreatic exocrine
secretion (27—29). A broad series of studies comparing the effects of atropine
and of the M1 receptor antagonist telenzepine on human pancreatic secretion
led to the conclusion, that the non-selective inhibition by atropine even is
predominantly achieved
via M1 receptors (29). More recently, analysis
of the rat acinar cell muscarinic receptor by polymerase chain reaction revealed
expression of both M1 and M3 subtypes (30). In addition, pharmacological blockade
of M1 receptors caused a significantly greater inhibition of amylase secretion
in isolated pancratic acinar cells than blockade of the M3 receptors (30).
Since at least three pharmacologically distinct subtypes (M1, M2, M3) of the
muscarinic receptor do exist, the question emerged, which subtype is responsible
for the vagal cholinergic control of pancreatic exocrine secretion. Based upon
studies in which the M3 receptors were pharmacologically blocked, the M3 receptor
was considered as the typical muscarinic receptor of the acinar cell (23—26).
In-vivo studies in humans and dogs, however, revealed a physiological role of
M1 receptors in the control of pancreatic exocrine secretion (27—29). A broad
series of studies comparing the effects of atropine and of the M1 receptor antagonist
telenzepine on human pancreatic secretion led to the conclusion, that the non-selective
inhibition by atropine even is predominantly achieved via M1 receptors (29).
More recently, analysis of the rat acinar cell muscarinic receptor by polymerase
chain reaction revealed expression of both M1 and M3 subtypes (30). In addition,
pharmacological blockade of M1 receptors caused a significantly greater inhibition
of amylase secretion in isolated pancratic acinar cells than blockade of the
M3 receptors (30).
M1 receptors and pancreatic bicarbonate secretion
The stimulation of pancreatic bicarbonate secretion by intraduodenal amino acids
such as tryptophan has been well established (13, 15, 35). However, tryptophan
does not increase plasma secretin concentrations over basal levels (35), indicating
the existence of other pathways that stimulate pancreatic bicarbonate secretion.
Telenzepine was found to significantly decrease the pancreatic bicarbonate response
to intraduodenal tryptophan in conscious dogs up to total abolition (18, 22,
28) (Fig. 1). Thus, intraduodenal amino acids stimulate bicarbonate secretion
in part
via M1 receptors. This mechanism
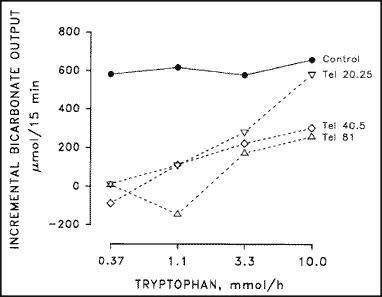 |
Fig. 1. Effect of different doses of telenzepine
(Tel; nmol/kg/h iv.) on the incremental pancreatic bicarbonate response
to graded loads of intraduodenal tryptophan. Results are means of 6
dogs. Data are adapted from Niebergall-- Roth et al. (22).
|
seems to play a major role in the mediation of the bicarbonate response to low
loads of intraduodenal amino acids, since the inhibitory effect of telenzepine
was greater when low loads of tryptophan were given (
Fig. 1).
M1 receptors and pancreatic enzyme secretion
During secretin infusion (in order to stimulate pancreatic fluid secretion),
telenzepine significantly decreased canine pancreatic enzyme output by up to
85% (18, 36) (
Fig. 2). Since secretin does not stimulate pancreatic enzyme
secretion in dogs (18, 28, 36), this finding suggests that M1 receptors are
important mediators of basal pancreatic enzyme secretion. The observation that
telenzepine still depresses basal enzyme output after truncal vagotomy (18)
(
Fig. 2) suggests that the basal cholinergic tone of the pancreas does
not significantly depend on the integrity of the vagus nerves but can be mainly
ascribed to the intrinsic cholinergic nerves.
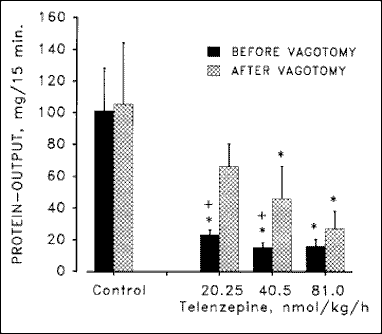 |
Fig. 2. Effect of different doses of telenzepine
on pancreatic protein output during intravenous infusion of secretin
(20.5 pmol/kg/h) before and after truncal vagotomy. Results are means
± SEM of 6 dogs. *p <0.05 vs. control. +p
< 0.05 before vs. after vagotomy. Data are adapted from Niebergall-Roth
et al. (18).
|
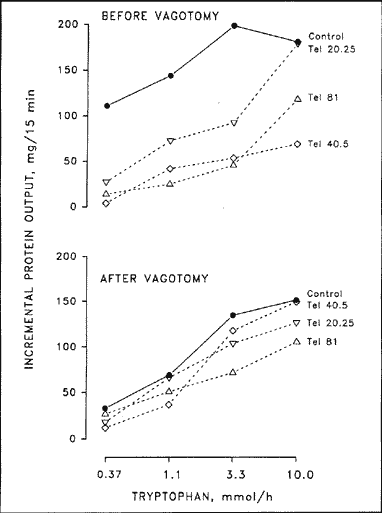 |
Fig. 3. Effect of different doses of telenzepine
(Tel; nmol/kg/h iv.) on the incremental pancreatic protein response
to graded loads of intraduodenal tryptophan before and after truncal
vagotomy. Results are means of six dogs. Data are adapted from Niebergall-
Roth et al. (18).
|
The involvement of M1 receptors was confirmed by studying the effect of telenzepine
on the latency of pancreatic amylase secretion after a rapid intraduodenal bolus
application of tryptophan or sodium oleate (37). In this study, telenzepine
increased the latency of amylase response to tryptophan and oleate by more than
10-fold (Table 1), indicating that enteropancreatic reflexes stimulate pancreatic
enzyme secretion via activation of M1 receptors.
Table 1. Effect of telenzepine
and L-364,718 on latency (in sec., unless otherwise stated) of pancreatic
amylase response to intravenous (iv.) and intraduodenal (id.) stimulants. |
|
Caerulein, 7.4 pmol/kg iv. |
L-Tryptophan, 1 mmol id. |
Sodium oleate, 3 mmol id. |
Control |
28 ± 4 |
17 ± 7# |
16 ±5# |
Telenzepine, 81 nmol/kg iv. |
30 ± 5 |
178 ± 116*# |
208 ± 121*# |
L-364,718, 0.1 mg/kg iv. |
>10 min. |
>10 min. |
>10 min. |
|
Data are means ± SEM of 6 dogs. *p < 0.05 vs. control;
#p < 0.05 vs. caerulein. Data are adapted
from Niebergall-Roth et al. (37). |
NEUROHORMONAL INTERACTIONS
Besides cholinergic nerves, the hormone CCK is
considered as the most important mediator of postprandial pancreatic exocrine
secretion, particularly pancreatic enzyme output. To investigate possible interactions
between cholinergic M1 receptors and CCK, we have studied the effects of telenzepine
in dogs during blockade of CCK-A-receptors by L-364,718 (MK-329, devazepide).
L-364,718 is an extremely potent competitive CCK antagonist with no intrinsic
activity and a high specifity for peripheral CCK-A-receptors (38).
Exogenous stimulation of the pancreatic protein output with the CCK analogue
caerulein is not affected by telenzepine (18, 36) (
Fig. 4). This is in
accordance with earlier findings that in dogs neither atropine nor vagotomy
had any significant effect on pancretic secretory response to exogenous CCK
or caerulein (15, 39, 40). In addition, L-364,718 depressed the canine pancreatic
protein response to tryptophan irrespective of the integrity of the vagus nerves
(18) (
Fig. 5). Thus, endogenously released CCK controls canine pancreatic
enzyme output at least in part by a pathway that does not involve M1 receptors,
probably as a classical humoral factor acting at acinar CCK receptors.
 |
Fig. 4. Effect of different doses of telenzepine
(Tel; nmol/kg/h iv.) on the incremental pancreatic protein response
to graded doses of intravenous caerulein. Results are means of 6 dogs.
Data are adapted from Niebergall-Roth et al. (36).
|
Obviously, this mechanism seems to represent a species particularity. In humans,
the stimulatory effect of physiological doses of exogenous CCK or caerulein
was almost completely inhibited by atropine (41—43), suggesting that exogenous
CCK at physiologic concentrations stimulates pancreatic secretion by interaction
with the cholinergic system. In rats, truncal vagotomy, atropine, hexamethonium
and the sensory neurotoxin capsaicin completely abolished the pancreatic protein
response to exogenous CCK given in a dose that produced postprandial plasma
concentrations (44). Thus, in rats the stimulatory action of exogenous CCK in
a physiologic dose on pancreatic secretion is mainly mediated via capsaicin-sensitive
vagal sensory afferents originating from the gastroduodenal mucosa (44).
Besides the action of CCK as a classical humoral factor, in dogs also experimental
evidence exists for an interaction between the cholinergic system and the hormone
CCK. The inhibition of the pancreatic protein response to intraduodenal tryptophan
caused by combination of telenzepine and L-364,718 was significantly greater
than the sum of the inhibitory effects of each antagonist when given alone.
In particular, the combination of the two antagonists in very low doses that
had no significant effects on protein output when given separately, totally
abolished the protein response when given together (18) (
Fig. 6). This
finding indicates that in dogs — in addition to directs effects of CCK on the
pancreatic acinar cell — a mechanism of potentiative interaction exists between
cholinergic nerves ending on M1 receptors and CCK. In addition, L-364,718 was
shown to interrupt the enteropancreatic reflex mediating the early amylase response
to intraduodenal bolus application of tryptophan and oleate (37), indicating
that the activation of (possibly vagal) CCK receptors is essential to run this
reflex.
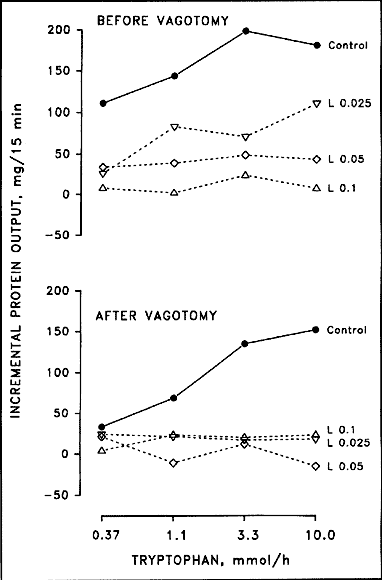 |
Fig. 5. Effect of different doses of L-364,718
(L; mg/kg/h iv.) on the incremental pancreatic protein response to graded
loads of intraduodenal trypotphan before and after truncal vagotomy.
Results are means of 6 dogs. Data are adapted from Niebergall- Roth
et al. (18).
|
The question, where the interaction between the cholinergic system and CCK in
the dog occurs, needs still to be answered. Firstly, CCK and cholinergic nerves
may interact at the level of the acinar cell in as much a threshold of cholinergic
input conditions the pancreatic secretory response of the acinar cell to hormonal
acting CCK. However, this hypothesis is incompatible with the observation, that
in dogs neither vagotomy, nor atropine, nor telenzepine alters the pancreatic
protein response to exogenous CCK or caerulein in dogs (13, 18, 45). Secondly,
CCK may activate cholinergic vagal neurons and thus modulate the intrapancreatic
neurons. An anatomical basis is provided by the discovery of type A receptors
for CCK on afferent vagal fibers (46).
An intriguing hypothesis that integrates both — humoral and vagally mediated
— pathways of CCK in the dog was provided by Grossman (cited in

Fig. 6. Effect of different doses of telenzepine (Tel; nmol/kg/h
iv.), L-364,718 (L; mg/kg/h iv.), or both on the incremental pancreatic
protein response to graded loads of intraduodenal tryptophan. Results
are means of 6 dogs. Data are adapted from Niebergall-Roth et al.
(18). |
(47)). Intraduodenal infusion of nutrients would release CCK from endocrine
cells of the duodenum. When low loads of nutrients were infused, only small
amounts of CCK would be released, that would not be discharged into the circulating
blood but would diffuse across the intracellular space to the nervous CCK receptor
and initiate a vago-vagal reflex. When high doses of nutrients were given intraduodenally,
greater amounts of CCK would be released from the intestinal endocrine cells
and discharged into the bloodstream and could thus stimulate pancreatic protein
output by acting at the acinar CCK receptor. The higher treshold for hormonal
than for nervous stimulation by CCK would explain the observation that in dogs
L-364,718 inhibited the protein reponse to low and high amounts of intraduodenal
nutrients, whereas telenzenzepine was mainly effective for inhibiting the response
to low amounts of nutrients (18, 28, 36) (
Figs. 3 & 5). Furthermore,
this mechanism would account for the finding that L-364,718 inhibits the pancreatic
protein response even to low loads of intestinal amiono acids, that do not cause
measurable elevation of plasma CCK (18).
INTERACTIONS BETWEEN CHOLINERGIC AND NONCHOLINERGIC
NERVES
Although several neurotransmitters found in pancreatic
nerve cell bodies or fibers can stimulate or inhibit pancreatic exocrine secretion
under distinct experimental conditions (
Table 2), the physiological significance
of most of them in the neural control of the exocrine pancreas needs to be further
evaluated. The most likely candidates are nitric oxide (NO), serotonin (5-HT)
and the neuropeptides vasoactive intestinal polypeptide (VIP), pituitary adenylate
cyclase activating polypeptide (PACAP) and gastrin releasing polypeptide (GRP)
(48)..Experimental evidence exists that nitrergic, serotonergic and GRP-containing
nerves modulate the cholinergic control of pancreatic exocrine secretion.
Table 2. Non-adrenergic non-cholinerigig
neurotransmitters present in the pancreas and their effect on pancreatic
exocrine secretion. |
Stimulatory neurotransmitters |
Inhibitory neurotransmitters |
|
Nitric oxide (NO) |
Serotonin (5-HT) |
Serotonin (5-HT) |
Calcitonin gene related peptide (CGRP) |
Vasoactive intestinal polypeptide
(VIP) |
Enkephalines |
Pituitary adenylate cyclase
activatingpolypeptide (PACAP) |
Galanin |
Gastrin releasing polypeptide (GRP) |
Neuropeptid Y (NPY) |
|
|
Nitric oxide (NO)
The gas NO fulfills several characteristics of a neurotransmitter in that it
is released from nerves and affects the function of effector cells. However
it is not preformed or stored in presynaptic cells like other transmitters.
The significance of NO for the regulation of pancreatic exocrine secretion has
been established by several studies in humans and animals (for review see (49)).
In conscious dogs, the inhibitory effect of the NO synthase inhibitor N
G-nitro-L-arginine
(L-NNA) was most pronounced after vagal stimulation of the pancreas by sham
feeding (50) or 2-deoxy-D-glucose induced neuroglycopenia (49, 51). These findings
suggest that the regulatory effect of NO on pancreatic secretion is related
to a vagal pathway.
Serotonin (5-HT)
The serotonergic nerve fibers found in the pancreas
are probably of enteric origin (20). The axons of the serotonergic enteropancreatic
neurons appear to form inhibitory axo-axonic synapses in the pancreas (52).
5-HT inhibits veratridine-mediated amylase secretion; thus the targets of the
serotonergic enteropancreatic innervation are likely to include the cholinergic
axons that innervate acinar cells. This effect of 5-HT was blocked by the 5-HT
1P
receptor antagonist, N-acetyl-5-hydroxytryptophyl-5-hydroxytryptophanamide (5-HTP-DP).
This observation suggest that serotonergic nerves inhibit pancreatic enzyme
secretion via presynaptic 5-HT1P receptors on cholinergic neurons (52).
Gastrin releasing polypeptide (GRP)
The amphibian peptide bombesin, its mammalian structural
counterpart GRP, and its C-terminal decapeptide neuromedin C, respectively,
strongly stimulate.pancreatic fluid, bicarbonate and enzyme secretion in humans,
pigs, dogs, and rats (53—57). Electrical field stimulation evoked release of
GRP in isolated perfused pig and rat pancreas (54, 58). In the isolated perfused
porcine pancreas, atropine inhibited the enzyme response to exogenous GRP, suggesting
that acetylcholine somehow sensitizes the acinar cell to the effects of GRP.
Another possiblity is that, in addition to acting directly at the acinar bombesin/GRP
receptor, GRP-containing nerves modulate pancreatic exocrine secretion through
stimulation of acetycholine release from postganglionic cholinergic fibers.
This hypothesis is supported by the findings that tetrodotoxin inhibited GRP
stimulated amylase release in rat pancreatic lobules (57) and that bombesin
had no effect on enzyme output from dog pancreatic acini (59).
FUTURE RESEARCH PERSPECTIVES
Despite an increasing knowledge about the function
of pancreatic innervation several unanswered questions still remain.
Regarding the cholinergic component in the control of pancreatic exocrine secretion,
the study of the effects of M3 receptor antagonists in vivo could clarify the
physiological role of acinar M3 receptors. The relative contribution of M1 and
M3 receptors to the cholinergic control of pancreatic exocrine secretion is
another intriguing question and could be answered by the comparison of the effects
of M1 and M3 receptor antagonists under distinct experimental conditions.
The function of the enteropancreatic innervation still remains hypothetical.
Further studies revealing the nature of the enteric stimuli that selectively
activate either the excitatory or the inhibitory components of the enteropancreatic
innervation will provide the needed insight into the function of this system.
Now that neurochemical investigations have revealed a number of peptides and
other neuroactive substances in the nerve cell populations of the pancreas,
subsequent studies should investigate the physiological significance of these
substances as neurotransmitters of these cells. In addition, further investigation
of interaction pathways between these neurotransmitters could contribute to
the understanding of the integration and/or summation of vagal, sympathetic,
enteric and intrinsic pancreatic signals.
Finally, attention should be paid to the role of the pancreatic innervation
in pathophysiological processes. Future studies are necessary to establish whether
and how pancreatic neurotransmission is altered or disrupted unter pathophysiological
conditions. A more comprehensive perspective of the neural mechanisms associated
with such processes will lead to a better understanding of pancreatic disease.