The rats used in the laboratories can be catalogued into inbred and outbred strains. Common inbred strains include the Brown Norway, Lewis, Fischer and F344. Common outbred stocks are Wistar, Long-Evans and Sprague-Dawley. Mean life-span of outbred rats, like Sprague-Dawley rats, is 1000 days (2.7 years) for males and 1300 days (3.6 years) for females. They double their body weight in the first 5 days after birth. Pups grow rapidly and at weaning the average body weight is about 45 g (1). Growth continues with maximum weight attained by 250-300 days. Although Sprague-Dawley rats will continue to grow throughout life, most of this adult "growth", is due to fat deposition. Rats have a well-defined diurnal rhythm, being active during the dark and sleeping and resting during the light hours. Feeding occurs at night and digestion during the early daylight hours. Laboratory rats are normally confined to cages that markedly restrict their physical activity, and therefore the resting energy expenditure accounts for 90% of the total daily energy expenditure, and daily physical activities only account for the remaining 10% (2-4).
In humans, the concepts of overweight and obesity are commonly assessed by using
body mass index (BMI), defined as the weight in kilograms divided by the square
of the height in meters (kg/m
2). A BMI over 25
kg/m
2 is defined as overweight, and a BMI of over
30 kg/m
2 as obese. People with a BMI below 18.5
kg/m
2 tend to be underweight. In rodents, like
rats and mice, the height and length are almost similar within the strains,
therefore body weight itself is a reliable index for being overweight. Analogous
to the human, obese rats are characterized as being overweight secondary to
an increased fat compartment.
Effective weight management for individuals and groups at risk of developing obesity involves a range of long-term strategies. These include prevention, weight maintenance, management of co-morbidities and weight loss. It has been commonly recommended to promote healthy eating behaviors by cutting the amount of fat and sugar contents in the diet (5). Weight loss treatments include diets, drugs, physical training, and surgery, namely bariatric or obesity surgery. So far, only bariatric surgery has demonstrated a long-term therapeutic effect. The common surgical procedures include i) restrictive operations such as vertical banded gastroplasty, silastic ring gastroplasty and gastric banding; ii) malabsorptive operations including variations of the intestinal bypass; and iii) combined operations which utilize both restriction and malabsorption including variations of short-limb, long-limb, or distal gastric bypass and biliopancreatic diversion. The current standard for bariatric surgery is the Roux-en-Y gastric bypass. In a worldwide survey, gastric bypass was performed in 65% of all bariatric surgeries (6-10).
The role of the stomach in regulating food intake and consequently body weight
has been well recognized, because it is generally thought that the stomach functions
as the primary source of satiety. It also functions as an acid resistent compartment
where food is mixed with gastric enzymes and acid (HCl). In addition, the stomach
produces various kinds of peptides/hormones (11, 12). There are at least 5 distinct
endocrine cell types in the rat stomach: 1) enterochromaffin-like (ECL) cells
with closed morphology in the oxyntic mucosa (13, 14), 2) G cells with open
morphology in the antral mucosa (15), 3) D cells with open morphology in the
antrum and closed morphology in the oxyntic mucosa (16, 17), 4) closed-type
enterochromaffin (EC) cells in the antral and oxyntic mucosa (18), 5) closed-type
A-like cells in the antral and oxyntic mucosa (19-23). A common characteristic
of these endocrine cells is storage of chromogranin A and its fragment pancreastatin
(24-27) (
Table 1).
Table 1. Endocrine cell types and their products in rat stomach. |
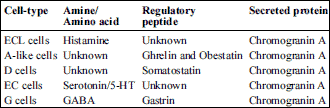 |
During the past years, we have studied the physiological mechanisms of the regulation of body weight development and feeding behavior in rat models for gastric bypass, gastrectomy, and high-fat diet-induced obesity. Our results and suggestions are summarized as follows.
WEIGHT LOSS AFTER GASTRIC BYPASS SURGERY IS
NOT
CAUSED BY EARLY SATIETY, REDUCED FOOD INTAKE,
AND/OR BY IMPAIRED NUTRIENT ABSORPTION
It is a general belief that there is a direct link between food intake and body weight. Countless studies have been conducted to understand the complex central and peripheral networks that regulate food intake in order to identify potential targets for effective anti-obesity therapy. One would intuitively expect that restriction of food intake will achieve body weight loss. Unfortunately, it has not been the case in the long-term for overweight people and for people suffering from obesity. The excellent clinical outcome after gastric bypass reduces the stomach to a small pouch and thereby creates a signaling for an earlier satiety with a subsequent reduction in food intake. Other consequence of the gastric bypass includes malabsorption by creating a short gut syndrome and/or by accomplishing distal mixing of bile and pancreatic juice with ingested nutrients thereby reducing absorption.
Long-Evans rats have often been used in obesity studies because one colony (named
Otsuka Long-Evans Tokushima Fatty, OLETF) develops spontaneously mild obesity
and diabetes due to a deficiency of CCK1 receptor (28). We have performed gastric
bypass surgery in male adult Long-Evans rats at body weight of 600 g (not specifically
OLETF). As expected, the rats lost about 30% of their body weight 5 months after
the surgery, which was likely accounted for by a low fat mass without affecting
the fat-free mass (
Fig. 1). Surprisingly, these rats did not have less
food intake than sham-operated controls (29). These results have been reproduced
3 times (3, 6, or 8 weeks, postoperatively) in Sprague-Dawley rats at body weight
of 600 g (30, 31). Moreover, satiety ratio was neither increased during daytime
nor night time, neither short-term (3 weeks) nor long-term (3.5 months) after
gastric bypass (30).
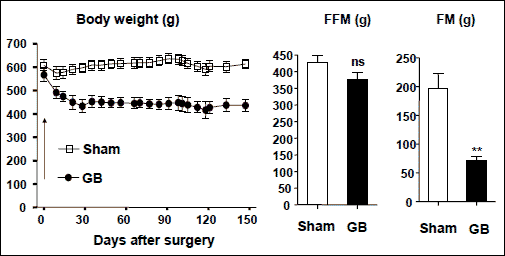 |
Fig. 1. Body weight, fat-free mass (FFM) and fat-mass (FM) in male Long-Evans rats after sham-operation or gastric bypass (GB). FFM and FM were measured during the last week of the study. ns- not significant **, p<0.01. |
Most gastric bypass surgeries are performed clinically with the so-called Roux-en-Y
procedure, which was introduced by Cesar Roux in 1897. Roux-en-Y gastric bypass
is believed to induce malabsorption by bypassing a long limb of the small bowel.
In order to eliminate the involvement of the small bowel, we compared the body
weight loss after total gastrectomy, stomach bypass (anastomosis of the esophagus
and duodenal bulb), or micro-gastric bypass (anastomosis of the esophagus and
the proximal jejunum). All these operations reduced body weight to a similar
degree (
i.e. 20-30%) (29). For comparison, the weight loss was ~25% after
Roux-en-Y gastric bypass (32). Hence, we suggest that the stomach
per se
is responsible for weight loss after gastric bypass (including Roux-en-Y procedure).
This conclusion is further supported by an unchanged energy density in the feces
after either gastric bypass or gastrectomy (30, 31).
It appears that the weight loss after gastric bypass is not due to the reduced food intake nor general malabsorption. We propose alternative explanations, including increased energy expenditure and/or reduced meal size after gastric bypass. Indeed, gastric bypass increased energy expenditure (expressed as kcal/h/100 g body weight) and decreased transiently meal size compared with age-matched sham-operated controls (30, 31).
CONTROL OF FOOD INTAKE IS INDEPENDENT OF THE
FOOD
RESERVOIR FUNCTION OF THE STOMACH, BUT PROBABLY
RELATED TO THE ENDOCRINE FUNCTION OF THE STOMACH
The rationale behind obesity surgery has been to reduce the stomach size for restricting food intake or bypass the stomach and part of intestine to induce malabsorption, which might not be like (see above). It has been suggested that the mechanisms underlying the body weight loss after gastric bypass may involve gastric hormones, since the stomach produces large amounts of various hormones, including ghrelin, obestatin, leptin, gastrin, and pancreastatin, in response to food intake (11, 12, 20, 33-37). One would expect 1) that removal of food reservoir by gastrectomy or gastric bypass would reduce food intake and 2) that elimination of the gastric hormones by gastrectomy or elimination of food stimulus by gastric bypass would alter appetite, food and water intake.
We have examined the effects of total gastrectomy vs. gastric bypass on eating behavior and gastric hormones. We found that total gastrectomy-induced body weight loss, but not gastric bypass-induced body weight loss, was associated with a reduced accumulated food intake, suggesting that the control of food intake was independent of the food reservoir function of the stomach, and furthermore that the surgical depletion of gastric hormones might be instrumental for the weight loss (29-31).
Exogenous ghrelin or ghrelin receptor agonist induce body weight gain by increasing
food intake (33, 38). One might predict that ghrelin depletion by gastrectomy
could be associated with a low food intake, leading to decreased body weight
gain. Indeed, gastrectomized rats have sustained low levels (20-50%) of ghrelin
(20, 31), and ghrelin treatment in gastrectomized mice partially reversed the
body weight reduction or increased body weight (37%) (38-40). The effects of
exogenous obestatin on food intake as originally reported (36) have been difficult
to reproduce by others (41, 42) under the same experimental conditions, most
likely because of instability and impurity of synthesized obestatin (42, 43).
Conversely, obestatin secretion was found to display an ultradian rhythmicity,
very similar to ghrelin and growth hormone secretion (44). In fact circulating
ghrelin and obestatin levels were not strictly correlated;
i.e. unchanged
ghrelin but reduced obestatin levels after gastric bypass (29) and increased
ghrelin but decreased obestatin after 24 hours of fasting (44). These findings
support the notion that obestatin is a physiologically relevant peptide and
not only a non-functional peptide (44). We have found that gastrectomy (which
induces hypoobestatinemia and hypoghrelinemia) was associated with increased
water consumption and drinking activity, particularly in daytime and seems not
to be associated with meal activity (31). These are in line with reports showing
that both ghrelin and obestatin were able to alter thirst mechanisms (45-47).
In addition, other gastric hormones including ECL-cell hormone (unidentified),
pancreastatin and leptin (48, 49), gut hormones (
e.g. CCK, peptide YY,
bombesin, glucagon-like peptide 1), and orexins (50) may be involved.
GHRELIN AND OBESTATIN ARE CO-LOCALIZED WITH
AN UNKNOWN AMINE IN THE A-LIKE CELLS IN THE STOMACH
AND EXPRESSED IN THE ISLETS OF THE PANCREAS
Ghrelin has been extensively studied since its discovery, while obestatin has been questioned in terms of its physiological significance (51-53). In our study, we showed that ghrelin and obestatin were both produced and stored in the A-like cells in the stomach, which represent approximately 20-30% of the total endocrine cell population in this organ (54). We showed further the ontogenic development of obestatin and preproghrelin on one side and ghrelin on the other side, particularly two weeks after birth. Moreover, preproghrelin- and obestatin-immunoreactive cells were more numerous than ghrelin-immunoreactive cells in the stomach, suggesting that some of A-like cells do not contain ghrelin (54). In addition, this study confirms the existence of a new endocrine cell of the pancreatic islets and co-localization of ghrelin and obestatin in the peripheral region of the pancreatic islets, a distribution distinct from glucagon-producing A-cells, insulin-producing cells, and pancreatic polypeptide Y-producing cells (54). It will be of great interest to study the role(s) of ghrelin and obestatin in regulation of beta-cell function, development and proliferation.
Immuno-electron microscopy showed that preproghrelin, ghrelin and obestatin
were located in the dense cores of granules in A-like cells (54). It is generally
known that the electrochemical gradient across membranes of granules/secretory
granules provides energy for the transport of small transmitter molecules such
as serotonin and histamine through VMAT
1 and
VMAT2 (55-57). VMAT
1 is thought to be responsible
for uptake of serotonin, while VMAT
2 is responsible
for uptake of histamine and is inhibited by reserpine (57, 58). We found that
A-like cells expressed both VMAT
1 and VMAT
2
but contained neither serotonin nor histamine (54). Taken together with biological
synthesis of ghrelin and obestatin, we propose a life cycle of ghrelin and obestatin
in the A-like cells (
Fig. 2). In brief, the granules with a high-electron
dense core are formed as a result of protein condensation (with acidic pH optima).
When the granules migrate towards the periphery of the cell, they start to take
up amine from the cytosol by means of VMAT
1 or 2,
which is located in the granule membrane. The continued accumulation of amine
is associated with formation of a halo between the dense core and granule membrane,
which proceeds conjointly with posttranslational modification, leading to the
generation of ghrelin, obestatin and pancreastatin. As a consequence of stimulation
of the cell, these secretory granules will fuse with the cell membrane ultimately
to release ghrelin, obestatin, pancreastatin and monoamine by exocytosis.
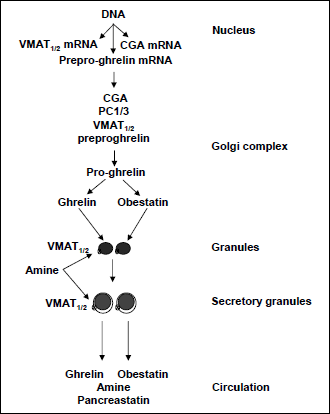 |
Fig. 2. Life cycle of secretory
granules in the A-like cells of stomach. The granular organelles develop
from the trans Golgi network, loaded with cargo, e.g. PC1/3, VMAT1or2,
chromogranin A (CGA), and preproghrelin (proceeding to proghrelin), and
take up amine from the cytosol by means of VMAT1or2,
which is located in the granule membrane. The continued accumulation of
amine is associated with formation of a halo between the dense core and
granule membrane. |
EXCESSIVE MEAL-SIZE IS RESPONSIBLE
FOR HIGH-FAT DIET-INDUCED OBESITY
It is a common belief that obesity is result of overnutrition and lack of exercise.
High-fat diet has often been used successfully for modeling the metabolic disorders
of human obesity in rodents, which enhances the belief that the development
of obesity is due to excess energy intake. Moreover, an extension of this belief
is that the aim of obesity surgery (
e.g. gastric bypass) is to reduce
food intake and nutrient absorption. We have utilized the so-called Comprehensive
Laboratory Animal Monitoring System to analyze food intake in rats that underwent
gastric bypass. Unexpectedly, we have found that the weight loss after the surgery
was neither due to reduced food (normal diet) intake nor impaired nutrient absorption
(29-31). We began to question the common belief that food/calorie intake plays
a conclusive role in the development of obesity.
It was reported that juvenile rats (male, Sprague-Dawley, 4 weeks old) fed solid
high-energy food did not develop overweight but had excess fat deposition. When
the animals were fed with a complete, balanced, liquid-diet supplement, chocolate-flavored
Ensure in addition to either control normal diet or high-energy diet, or both,
the rats had an increased body weight gain as well as fat content (59, 60).
We have established a simple and effective procedure to induce obesity in rats,
i.e., the rats at 3 weeks of age (after weaning) were exposed to a mixture
of normal and high-fat diets (50:50) in forms of both food pellets and powders
(50:50) for 2 weeks and then turned to 100% high-fat diet (pellets and powders)
(61). Two groups of rats were fed with a standard high-fat diet (containing
60% fat in calories) vs. normal standard diet (10% fat in calories) for a time
period from 3 weeks of age (after weaning) to 43 weeks (adulthood). As a result,
the rats on high-fat diet were already overweight at 9 weeks of age and later
became obese, characterized by increased body weight and excess fat deposition
(
Fig. 3) (61).
 |
Fig. 3. Body weight, fat-free mass (FFM) and fat-mass (FM) in male Sprague-Dawley rats fed with either normal diet or high-fat diet. ** p<0.01, ns- not significant. |
We have shown that gastric bypass stunted the growth of young rats, and caused the loss of body weight (mainly fat mass) in adult rats as well as overweight (Long-Evens) rats (29). The reduced body weight was not associated with food intake or with ghrelin production (29-31). We have also showed that the high-fat diet-induced obesity was neither associated with increased food/calorie intake nor reduced energy expenditure (61). Interestingly, the rats on high-fat diet developed an altered eating behavior, characterized by rapid consumption of large portions of food from early an age, and later became obese (61). It is, therefore likely that the higher calorie intake per meal will lead to lower energy costs of incorporating dietary energy into the body. In addition, we observed hyperplasia of ghrelin-producing cells in the stomach, which conceivably enables a high production of this appetite hormone in response to a meal (61). The precise biological mechanisms underlying high-fat diet induced obesity and its relevance to humans still need to be further studied.
PREVENTIVE STRATEGIES FOR OBESITY EPIDEMIC:
MEAL SIZE
REDUCTION IN EARLY CHILDHOOD AND ADOLESCENCE,
AND ENERGY EXPENDITURE IN ADULTHOOD
Obesity prevention during infancy, childhood and adolescence is significantly important. Current obesity prevention interventions include at least: promoting breastfeeding, improving diet, reducing portion sizes, limiting availability of snack foods in schools and workplaces, reducing food advertising, and increasing physical activity. However, individual responsibility is insufficient and therefore prevention strategies for the obesity epidemic are have been a global failure (5). In order to determine whether there are causal links between origins of childhood and adulthood obesity and between caloric intake and obesity, randomized controlled trials should be conducted. However, such randomized trials in humans are ethically impossible. Not only the ethics but also the logistics for measuring the precise food and caloric intake, meal pattern, and energy expenditure for a continuous over the time periods required in infants, children and adults are almost impossible. Hence, epidemiological and animal studies are especially important for understanding the causal pathways of obesity and biological mechanisms of obesity development, leading to the development of evidence-based and practical prevention strategies for the growing obesity epidemic.
The obesity epidemic has been blamed on the modern life style, including food
consumptions, and physical activity. The Tackling Obesities - Future Choices
project report by the UK Foresight Programme claims that the technological revolution
of the 20
th century has led to weight gain becoming
unavoidable for the majority of the population, because our bodies and biological
makeup are out of step with our surroundings, and concludes that individual
responsibility is important but insufficient to tackle obesity on its own (5).
The Health Profile of England declares that this is a time to consolidate best
practice - by promoting breastfeeding, fruit and vegetable consumption, low
glycaemic-index diet, and smaller portion sizes - and to explore new interventions
which should be evaluated carefully to inform future practice (62). This so-called
best practice, targeting the modern life style, is unlikely to be efficient
because of too many targets and the limitations of human self-control over automatic
behaviors (63). We have shown that high-fat diet induced obesity in rats, like
humans (61). This is result of increased high energy intake per individual meal
(
Fig. 4) (61). Today in modern society, children (including infants)
usually have free access (and sometimes are encouraged) to consume large portion
of high-energy and high-fat food. Hence, it will be of great importance to conduct
epidemiological investigations and clinical trials to find the correlation between
individual meal size and body weight development during early periods of life
(early childhood and adolescent). This can easily be done by mothers if the
obesity prevention strategies focus on limiting children's dietary fat content
and meal size. Interestingly, it has been hypothesized that as-often-as-possible
breastfeeding favors the development of snacking during childhood, a habit which
in turn favors the development of obesity later (64). If these theories can
be supported by clinical trials, these methods will represent the best practice
for tackling the obesity epidemic.
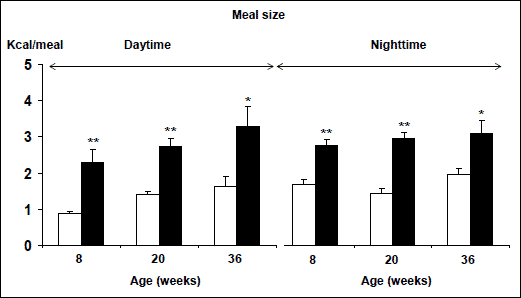 |
Fig. 4. Meal size during daytime and nighttime in male Sprague-Dawley rats measured at different ages. The rats were fed with either normal diet (white column) or high-fat diet (black column). *, **, p<0.05 or 0.01. |
POTENTIAL TARGETS FOR OBESITY TREATMENT:
ENERGY EXPENDITURE, FAT-CELL VOLUME AND TURNOVER
It is well known that aging is associated with decreased energy expenditure.
We have found that gastric bypass increased the energy expenditure compared
with age-matched sham-operated rats (30). Thus, we suggest that the mechanisms
underlying gastric bypass-induced body weight loss probably involve energy expenditure
rather than food intake, and moreover that the anti-obesity therapeutic strategies
should be more focused on increasing energy expenditure rather than on reducing
food intake. Indeed, drugs that aim to reduce body weight by increasing energy
expenditure have been explored. For instance, ß
3-adrenergic
receptor agonist (KRP-204) is at phase 2 trials (64).
We have also shown that the development of lean tissue and bone was impaired after gastric bypass (29). Hence, we do not recommend performing gastric bypass surgery for obese children and adolescents.
It has been demonstrated that the number of fat cells increases in childhood and adolescence, but levels off and remains constant in adulthood, and moreover that bariatric surgery results in a significant decrease in BMI and fat cell volume; however, it does not reduce the number of fat cells (65). This study might be interpreted as if one was overweight and then loses weight; one still has the capacity to store lipids because of having the same number of fat cells. This study provides an explanation for why it is so hard to keep weight off once it has been lost. Obese people who have already accumulated a large pool of fat cells in childhood and adolescence need to be treated by targeting the volume, if not the number, of their fat cells. A further challenge will be to seek drugs that target the mechanisms underlying fat-cell turnover.
Acknowledgements:
The studies conducted at the Department of Cancer Research and Molecular Medicine,
Norwegian University of Science and Technology (NTNU), Trondheim, Norway, and
were supported by grants from the Research Council of Norway, National Strategies
on Cancer Research, and St. Olavs' Hospital Foundation for Cancer Research,
Trondheim, Norway.
These studies have been included in a PhD thesis by Marianne Furnes at NTNU.
Conflicts of interest: None declared.
REFERENCES
- Pass D, Freeth G. The rat. ANZCCART News 1993; 6: 1-4.
- Ichikawa M, Fujita Y. Effects of nitrogen and energy metabolism on body weight in later life of male Wistar rats consuming a constant amount of food. J Nutr 1987; 117: 1751-1758.
- Ichikawa M, Kanai S, Ichimaru Y, Funakoshi A., Miyasaka K. The diurnal rhythm of energy expenditure differs between obese and glucose-intolerant rats and streptozotocin-induced diabetic rats. J Nutr 2000; 130: 2562-2567.
- Miyasaka K, Ichikawa M, Kawanami T, et al. Physical activity prevented age-related decline in energy metabolism in genetically obese and diabetic rats, but not in control rats. Mech Ageing Dev 2003; 124: 183-190.
- King D. Foresight report on obesity. Lancet 2007; 370: 1754.
- Mun EC, Blackburn GL, Matthews JB. Current status of medical and surgical therapy for obesity. Gastroenterology 2001; 120: 669-681.
- Solomon C, Dluhy RG. Bariatric surgery - quick fix or long-term solution? N Engl J Med 2004; 351: 2751-2753.
- Wolfe BM, Austrheim-Smith IT, Ghaderi N. Surgical treatment of obesity: pyloric electrical stimulation. Gastroenterology 2005; 128: 225-228.
- Martin LF. The evolution of surgery for morbid obesity. In Obesity Surgery, Martin LF (ed). New York, Mc Graw Hill, Medical Publishing Division, 2004, pp. 15-48.
- Pories WJ, Roth JS. Gastric bypass. In Obesity Surgery, Martin LF (ed). New York, Mc Graw Hill, Medical Publishing Division, 2004, pp. 213-225.
- Hakanson R, Sundler F. The Stomach as an Endocrine Organ. Amsterdam, Elsevier Science Publishers B.V., 1991.
- Dockray G. Gut endocrine secretions and their relevance to satiety. Curr Opin Pharmacol 2004; 4: 557-560.
- Hakanson R, Owman C. Concomitant histochemical demonstration of histamine and catecholamines in enterochromaffin-like cells of gastric mucosa. Life Sci 1967; 6: 759-766.
- Hakanson R, Bottcher G, Ekblad E, et al. Histamine in endocrine cells in the stomach. A survey of several species using a panel of histamine antibodies. Histochemistry 1986; 86: 5-17.
- Pearse AG, Bussolati G. The identification of gastrin cells as G cells. Virchows Arch A Pathol Pathol Anat 1972; 355: 99-104.
- Alumets J, Sundler F, Hakanson R. Distribution, ontogeny and ultrastructure of somatostatin immunoreactive cells in the pancreas and gut. Cell Tissue Res 1977; 185: 465-479.
- Canese MG, Bussolati G. Immuno-electron-cytochemical localization of the somatostatin cells in the human antral mucosa. J Histochem Cytochem 1977; 25: 1111-1118.
- Inokuchi H, Kawai K, Takeuchi Y, Sano Y. Immunohistochemical demonstration of EC cells in rat gastrointestinal tract. Histochemistry 1982; 74: 453-456.
- Date Y, Kojima M, Hosoda H, et al. Ghrelin, a novel growth hormone-releasing acylated peptide, is synthesized in a distinct endocrine cell type in the gastrointestinal tracts of rats and humans. Endocrinology 2000; 141: 4255-4261.
- Dornonville de la Cour C, Bjorkqvist M, Sandvik AK, et al. A-like cells in the rat stomach contain ghrelin and do not operate under gastrin control. Regul Pept 2001; 99: 141-150.
- Sakata I, Nakamura K, Yamazaki M, et al. Ghrelin-producing cells exist as two types of cells, closed- and opened-type cells, in the rat gastrointestinal tract. Peptides 2002; 23: 531-536.
- Rindi G, Necchi V, Savio A, et al. Characterisation of gastric ghrelin cells in man and other mammals: studies in adult and fetal tissues. Histochem Cell Biol 2002; 117: 511-519.
- Yabuki A, Ojima T, Kojima M, et al. Characterization and species differences in gastric ghrelin cells from mice, rats and hamsters. J Anatomy 2004; 205: 239-246.
- Fischer-Colbrie R, Lassmann H, Hagn C, et al. Immunological studies on the distribution of chromogranin A and B in endocrine and nervous tissues. Neuroscience 1985; 16: 547-555.
- Hawkins KL, Lloyd RV, Toy KA. Immunohistochemical localization of chromogranin A in normal tissues from laboratory animals. Vet Pathol 1989; 26: 488-498.
- Chen D, Monstein HJ, Nylander AG, et al. Acute responses of rat stomach enterochromaffinlike cells to gastrin: secretory activation and adaptation. Gastroenterology 1994; 107: 18-27.
- Norlen P, Curry WJ, Chen D, et al. Expression of the chromogranin A-derived peptides pancreastatin and WE14 in rat stomach ECL cells. Regul Pept 1997; 70: 121-133.
- Schwartz GJ, Whitney A, Skoglund C, et al. Decreased responsiveness to dietary fat in Otsuka Long-Evans Tokushima fatty rats lacking CCK-A receptors. Am J Physiol 1999; 277: 1144-1151.
- Stenstrom B, Furnes MW, Tommeras K, et al. Mechanism of gastric bypass-induced body weight loss: one-year follow-up after micro-gastric bypass in rats. J Gastrointest Surg 2006; 10: 1384-1391.
- Furnes MW, Tommeras K, Arum CJ, et al. Gastric bypass surgery causes body weight loss without reducing food intake in rats. Obes Surg 2008; 18: 415-422.
- Furnes MW, Stenstrom B, Tommeras K, et al. Feeding behavior in rats subjected to gastrectomy or gastric bypass surgery. Eur Surg Res 2008; 40: 279-88.
- Meguid MM, Ramos EJ, Suzuki S, et al. A surgical rat model of human Roux-en-Y gastric bypass. J Gastrointest Surg 2004; 8: 621-630.
- Kojima M, Hosoda H, Date Y, et al. Ghrelin is a growth-hormone-releasing acylated peptide from stomach. Nature 1999; 402: 656-660.
- Hakanson R, Ding XQ, Norlen P, et al. Circulating pancreastatin is a marker for the enterochromaffin-like cells of the rat stomach. Gastroenterology 1995; 108: 1445-1452.
- Cummings DE. Ghrelin and the short- and long-term regulation of appetite and body weight. Physiol Behav 2006; 89: 71-84.
- Zhang JV, Ren P-G, Avsian-Kretchmer O, et al. Obestatin, a peptide encoded by the ghrelin gene, opposes ghrelin's effects on food intake. Science 2005; 310: 996-999.
- Dun SL, Brailoiu GC, Brailoiu E, et al. Distribution and biological activity of obestatin in the rat. J Endocrinol 2006; 191: 481-489.
- Strassburg S, Anker SD, Castaneda TR, et al. Long-term effects of ghrelin and ghrelin receptor agonists on energy balance in rats. Am J Physiol Endocrinol Metab 2008; 295: E78-E84.
- Dornonville de la Cour C, Lindqvist A, Egecioglu E, et al. Ghrelin treatment reverses the reduction in weight gain and body fat in gastrectomised mice. Gut 2005; 54: 907-913.
- Lindqvist A, de la Cour CD, Hakanson R, et al. Ghrelin affects gastrectomy-induced decrease in UCP1 and beta3-AR mRNA expression in mice. Regul Pept 2007; 5: 24-28.
- Bresciani E, Rapetti D, Dona F, et al. Obestatin inhibits feeding but does not modulate GH and corticosterone secretion in the rat. J Endocrinol Invest 2006; 29: RC16-RC18.
- Nogueiras R, Pfluger P, Tovar S, et al. Effects of obestatin on energy balance and growth hormone secretion in rodents. Endocrinology 2007; 148: 21-26.
- De Spiegeleer B, Vergote V, Pezeshki A, et al. Impurity profiling quality control testing of synthetic peptides using liquid chromatography-photodiode array-fluorescence and liquid chromatography-electrospray ionization-mass spectrometry: the obestatin case. Anal Biochem 2008; 15: 229-234.
- Zizzari P, Longchamps R, Epelbaum J, et al. Obestatin partially affects ghrelin stimulation of food intake and GH secretion in rodents. Endocrinology 2007; 148: 1648-1653.
- Samson WK, White MM, Price C, et al. Obestatin acts in brain to inhibit thirst. Am J Physiol Regul Integr Comp Physiol 2007; 292: 637-643.
- Samson WK, Yosten GL, Chang JK, et al. Obestatin inhibits vasopressin secretion: evidence for a physiological action in the control of fluid homeostasis. J Endocrinol 2008; 196: 559-564.
- Hashimoto H, Fujihara H, Kawasaki M, et al. Centrally and peripherally administered ghrelin potently inhibits water intake in rats. Endocrinology 2007; 148: 1638-1647.
- Stenstrom B, Zhao CM, Tommeras K, et al. Is gastrin partially responsible for body weight reduction after gastric bypass? Eur Surg Res 2006; 38: 94-101.
- Zieba DA, Szczesna M, Klocek-Gorka B, et al. Leptin as a nutritional signal regulating appetite and reproductive processes in seasonally-breeding ruminants. J Physiol Pharmacol 2008; 59(Suppl 9): 7-18.
- Konturek SJ, Konturek JW, Pawlik T, et al. Brain-gut axis and its role in the control of food intake. J Physiol Pharmacol 2004; 55: 137-154.
- Kojima M, Kangawa K. Drug insight: the functions of ghrelin and its potential as a multitherapeutic hormone. Nat Clin Pract Endocrinol Metab 2006; 2: 80-88.
- Gourcerol G, St-Pierre DH, Tache Y. Lack of obestatin effects on food intake: should obestatin be renamed ghrelin-associated peptide (GAP)? Regul Pept 2007; 141: 1-7.
- Ceranowicz P, Warzecha Z, Dembinski A, et al. Pretreatment with obestatin inhibits the development of cerulein-induced pancreatitis. J Physiol Pharmacol 2009; 60: 95-101.
- Zhao CM, Furnes MW, Stenstrom B, et al. Characterization of obestatin- and ghrelin-producing cells in the gastrointestinal tract and pancreas of rats: an immunohistochemical and electron-microscopic study. Cell Tissue Res 2008; 331: 575-87.
- Schuldiner S, Shirvan A, Linial M. Vesicular neurotransmitter transporters: from bacteria to humans. Physiological Rev 1995; 75: 369-392.
- Liu Y, Edwards R. The role of vesicular transport proteins in synaptic transmission and neural degeneration. Annu Rev Neurosci 1997; 20: 125-156.
- Weihe E, Eiden LE. Chemical neuroanatomy of the vesicular amine transporters. FASEB J 2000; 14: 2435-2449.
- Zhao CM, Chen D, Lintunen M, et al. Effects of reserpine on ECL-cell ultrastructure and histamine compartmentalization in the rat stomach. Cell Tissue Res 1999; 295: 131-140.
- Archer ZA, Corneloup J, Rayner DV, et al. Solid and liquid obesogenic diets induce obesity and counter-regulatory changes in hypothalamic gene expression in juvenile Sprague-Dawley rats. J Nutr 2007; 137: 1483-1490.
- Archer ZA, Rayner DV, Rozman J, et al. Normal distribution of body weight gain in male Sprague-Dawley rats fed a high-energy diet. Obes Res 2003; 11: 1376-1383.
- Furnes MW, Zhao CM Chen D. Development of obesity is associated with increased calories per meal rather than per day. A study of high-fat diet-induced obesity in young rats. Obes Surg 2009; 19: 1430-1438.
- Editorial: Time to supersize control efforts of obesity. The Lancet 2007; 370: 1521.
- Cohen D, Farley TA. Eating as an automatic behavior. Prev Chronic Dis 2008; 5: 1-7.
- Arch JR. Beta(3)-Adrenoceptor agonists: potential, pitfalls and progress. Eur J Pharmacol 2002; 440: 99-107.
- Spalding KL, Arner E, Westermark PO, et al. Dynamics of fat cell turnover in humans. Nature 2008; 5: 783-787.