EXPOSURE TO SIXTY MINUTES OF HYPEROXIA UPREGULATES MYOCARDIAL HUMANINS IN PATIENTS WITH CORONARY ARTERY DISEASE - A PILOT STUDY
INTRODUCTION
Oxygen therapy is a standard of care for cardiac patients. Although being applied widely, surprisingly little is known about the cellular effects of oxygen therapy, in particular, of hyperoxia. The experimental studies performed more than a decade ago introduced the concept of preconditioning-like cardioprotection by hyperoxia (1). Pretreatment of animals with hyperoxia improved recovery of postischemic contractile function, reduced infarct size both in normal and atherosclerotic hearts, reduced incidence of ischemia-reperfusion induced arrhythmias and apoptotic cell death (2-6).
Despite of positive experimental results, translation of this concept into clinical medicine has had several drawbacks. Two subsequent trials on cardiac surgery patients did not show any protective effects of hyperoxia, applied either for 130 or 60 minutes before cardiopulmonary bypass (7, 8). There are several ways to explain these negative results. First, in case of modern myocardial protection techniques only minimal myocardial injury is caused by cardioplegic cardiac arrest, and there could be no need for activation of intrinsic protective mechanisms. Second, there is a possibility that the phenomenon of hyperoxia induced myocardial protection does not exist in humans at all.
In animals, short exposure to normobaric or hyperbaric oxygen induces changes in intracellular signalling, enzymes acitivity and gene expression. In the rat brain, activities of superoxide dismutase, catalase, glutathione peroxidase and glutathione reductase are increased (9). In the rat heart, hyperbaric oxygen enhances enzymatic activity and gene expression of catalase, thereby significantly reducing the infarct size after reperfusion (10). Achieved cardioprotection is related to redox-sensitive transcription factor NFκB (11), tumor necrosis factor receptor 1 - (12), opening of mitochondrial ATP-sensitive potassium channels (13), and mitogen activated protein kinases- and nitric oxide-dependant mechanisms (14). Most of these protective mechanisms seem to be universal as they are activated as a result of different conditioning stimuli (i.e classic ischemic preconditioning, postconditioning as well as remote ischemic preconditioning (15)). Whether all these changes occur in humans, is not known.
Detection of different triggering or signalling molecules is one possible approach to demonstrate adaptive changes induced by hyperoxia. But to study cellular signalling is difficult in a clinical setting - there always exists the possibility that some pathways remain out of scope, and studies with receptor antagonists or agonists are often not possible. Another approach would be to evaluate changes in myocardial gene expression. In particular, applying expression profile analysis on genome wide level by RNA sequencing analysis would allow detection of all possible changes related to hyperoxic pretreatment.
On that background, the present study was undertaken to describe whether a short exposure to hyperoxia induces changes in gene expression in the human myocardium, in patients with coronary artery disease. We hypothesized that 60 min exposure to hyperoxia is sufficient to induce overexpression of genes related to possible adaptive cardioprotection.
MATERIALS AND METHODS
Patients
The study design was approved by the Ethics Review Committee on Human Research of the University of Tartu. The investigation conforms with the principles outlined in the Declaration of Helsinki. Written informed consent was signed by all patients before the study. The study was performed in the departments of Cardiac Surgery of two tertiary care centres in Estonia - Tartu University Hospital and North Estonia Medical Centre. Adult patients scheduled for isolated primary elective coronary artery bypass grafting with at least 3 distal anastomoses were included and randomised into the control and hyperoxia groups (Fig 1). The exclusion criteria were as follows: 1) preoperative infusion of vasoactive or inotropic medications, 2) diabetes mellitus treated either with insulin or oral medications, 3) preoperative elevated troponin T, and 4) pulmonary disease.
All medications, except salicylates were allowed until the day of surgery.
Study protocol
After induction of anesthesia and intubation of the trachea, patients were randomly allocated to receive either 40% or > 96% oxygen for 60 minutes. The randomisation was performed by a phone call to a randmisation centre where method of permuted blocks was used. Thereafter the mixture of oxygen and air was adjusted until the beginning of cardiopulmonary bypass (CPB) to obtain arterial pO2 levels in the range of 100 – 150 mmHg. The fraction of oxygen in inspired gas mixture was continuously monitored by the gas analyser of the patient monitor. Arterial blood gases were analysed (Radiometer ABL 700 series, Radiometer Medical A/S, Copenhagen, Denmark) 10 and 60 minutes after randomisation. The patients were ventilated according to the protocol until the cannulation for CPB during which a tissue sample was harvested from the right atrial appendage for gene analysis.
Standardized intravenous anesthesia with propofol, fentanyl and midazolam was used. The patients were followed in the intensive care unit for 48 postoperative hours.
Tissue samples and gene analysis
Myocardial tissue samples were immediately immersed in RNALater, kept at +4°C and thereafter stored at –80°C for later gene expression analysis.
For RNA extraction the tissue was homogenized using mortar and liquid nitrogen. Total RNA was extracted from heart tissue with the mirVana miRNA Isolation Kit (Thermo Fisher Scientific Inc, Waltham, MA, USA) according to the manufacturers' protocol. During the purification DNase I treatment was performed on column according to manufacturers' protocol (Qiagen Sciences, Germantown, MD, USA). The RNA quality was assessed using Agilent 2100 Bioanalyzer and the RNA 6000 Nano kit (Agilent Technologies Inc. CA, USA). The RIN (RNA integrity number) for all samples was at least 7.45 ng of total RNA. RNA was amplified with Ovation RNA-Seq System V2 Kit (NuGen, Emeryville, CA, USA) and the output double stranded DNA was used to prepare SOLiD 5500 W System DNA fragment library according to manufacturers’ protocols (Thermo Fisher Scientific Inc, Waltham, MA, USA). The barcoding primers in library preparation and fragment sequencing chemistry was applied (75 bp in forward directions). In case of 12 samples per flowchip lane approximately 33 million mappable reads were expected per one sample, which would be enough for successful whole transcriptome expression pattern analysis.
Statistical analysis
Statistical analysis was performed in statistical environment R. Non-normalized raw counts were used for the EdgeR package to perform differential gene expression analysis after quality control of the samples. EdgeR performs model-based normalization, estimates dispersions and applies modelling. EdgeR is very flexible tool for RNAseq data analysis to find differentially expressed genes (16, 17). We used groupwise comparison between two treatment groups where negative binomial fitting was followed by exact test for determining differential expression. False discovery rate (FDR) adjustment was used for multiple testing correction (18). The FDR threshold for statiscal signifcance was set to 0.1.
Patient data were compared with Fisher´s exact test or Student t-test as appropriate.
Functional annotation of differentially expressed genes
The functional analysis of a gene network was used to identify the biological functions that are most significantly related to the molecules in the network. To define the functional networks of differentially expressed genes, data were analyzed by using the Ingenuity Pathway Analysis (IPA, Ingenuity Systems, www.ingenuity.com) that calculates a significance score (network score) for each network. This score indicates whether the likelihood that the assembly of a set of focus genes in a network could be explained by random chance alone (e.g., score of 2 indicates that there is a chance of 1 in 100 that the focus genes are together in a network due to random chance). A data set containing the gene identifiers and their corresponding fold change (log2) values were uploaded into the IPA software. Each gene identifier was mapped to its corresponding gene object in the Ingenuity Pathways Knowledge Base to identify molecules whose expression was significantly differentially regulated (focus genes or Networks Eligible molecules). These focus genes were overlaid onto a global molecular network developed from information contained in the Ingenuity Knowledge Base. Networks of these focus genes were then algorithmically generated based on their connectivity. A network represents the molecular relationships between genes or gene products, which are represented as nodes, and the biological relationship between two nodes is represented as an edge line. All edges are supported by at least one reference from the literature, or from canonical information stored in the Ingenuity Pathways Knowledge Base. Fold change difference threshold for the functional analysis was set on 2 (log2 transformed).
RESULTS
Patient demographics and clinical data
Patients' characteristics are given in Table 1. There were no inter-group differences regarding preoperative medications and operational data, but patients in the hyperoxia group were slightly younger. Also, the clinical course in the postoperative period as well as postoperative release of troponin T, decreased values of which could be interpreted as decreased myocardial damage after hyperoxic exposure, did not differ between groups.
Mean 'wash-out' time after 60 min exposure to > 96 % oxygen until the collection of tissue samples was 51 (13 – 145) min.
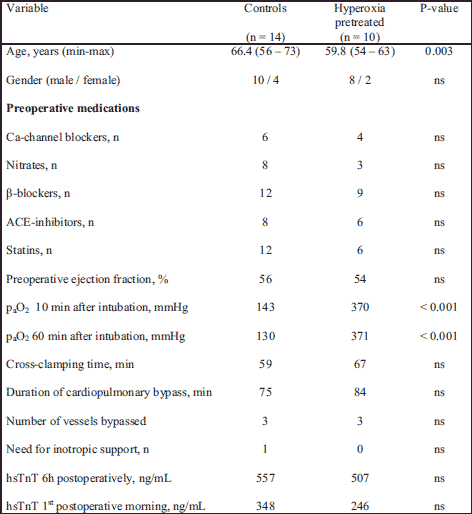
![]() |
Fig. 1. Flow diagram of the study. |
Gene expression
The RNA data can be found in GEO repository - GSE66249. By genome wide chip analysis 23100 genes were identified in the myocardial samples. Hyperoxic exposure caused significant changes in expression of 20 genes (Figs. 2 and 3). Most remarkable genes are two different humanins from the 10-member humanin family - MTRNR2L2 and MTRNR2L8.
![]() |
Fig. 2. Genes with significant changes in expression after pre-treatment with hyperoxia. Normalized counts of the 20 significantly changed gene expression values are illustrated, boxplots depict the different study groups (black dots for hyperoxia group, white dots for controls). Genes are sorted based on their FDR value. MTRNR2L2 and MTRNR2L8 are humanin 2 and humanin 8. Negative value of LogFC indicates supression, positive up-regulation of gene. |
![]() |
Fig. 3. Hierarchical clustering (heat map) depicting expression profiles of differentially regulated genes in the cardiomyocytes of patients from hyperoxia (red, 100) and control (green, 40) groups. Gene expression levels are depicted as color variation from dark blue (high expression) to pale green (low expression). The color in each cel of the figure displays the level of expression for each gene (rows) of each individual sample (columns). |
No significant differences in gene expression between men and women were found.
Ingenuity Pathway Analysis
To uncover potential regulatory network invoked during hyperoxic treatment, network maps were constructed using Ingenuity Pathway Analysis software. This helps to reveal potential common patterns in the gene expression activation, subject to regulation by the genes showing the highest differential expression after pre-treatment with > 96% oxygen. Gene network analysis allows to find and to identify functional consequence of this activation. Two different approaches were used. First, genes with the lowest FDR values (e.g. with the highest statistical confidence) were analyzed. Functionally the most relevant activated biochemical network was named 'cell survival', where upregulation of ESCO2 and S100A8 was evident (Fig. 3). As a second approach, we analyzed functional impact of genes with the highest-fold changes. This analysis indicated upregulation of several networks, from which the network 'cell-to-cell signalling and interaction' was functionally the most relevant (Figs. 4 and 5).
![]() |
Fig. 4. Network map illustrating the activation of cell survival network as a response to the stimulation with hyperoxia. Red colour depicts up-regulation of the gene, green colour depicts down-regulation of the gene. Small bars by the symbol indicate quantitative value F up- or down-regulation. |
![]() |
Fig. 5. Network map illustrating the activation of cell to cell signaling and interaction network in response to stimulation with hyperoxia. Red colour depicts up-regulation of the gene, green colour depicts down-regulation of the gene. Small bars by the symbol indicate quantitative value F up- or down-regulation. |
DISCUSSION
Exposure to > 96% oxygen for 60 minutes significantly changes the expression of 20 different genes, including upregulation of two different humanins in the heart of the patients with coronary artery disease. This is the first time to demonstrate that short-lasting breathing of hyperoxic gas mixture enables significant cellular response in the human heart.
Upregulation of two different humanins from a 10-member humanin family is of particular interest. Humanins, first discovered in 2001 (19), are small anti-apoptotic peptides originating from the human mitochondrial genome. By now, the cytoprotective effect of humanins has been established in a number of different stress situations, including oxidative stress (20).
In the murine model, humanins ameliorate the effects of left coronary occlusion. Pretreatment or treatment immediately following the artery occlusion reduced infarct size and loss of cardiac function, and decreased the apoptosis after myocardial ischemia and reperfusion (21). Humanin also has a protective effect on endothelial function, via modulating oxidative stress and apoptosis in the developing atherosclerotic plaque (22).
Humanins are expressed also in the human vasculature, in the endothelial cell layer of different blood vessels and in atherosclerotic plaques (23, 24). Exogenous addition of humanin analogue to endothelial cell cultures has shown to be effective against Ox-LDL-induced oxidative stress (23). In patients undergoing coronary angiography preserved coronary endothelial function has been associated with higher systemic humanin levels (25).
Interestingly, Ingenuity Pathway Analysis did not detected involvement of humanins (MTRNR2L2 and MTRNR2L8) in any of the cell survival networks (Figs. 4 and 5). This can be explained by the limited amount of available information about the humanins. On the other hand, ESCO2, the gene what was induced by hyperoxia significantly and with high-fold change (2.8 times induced), belongs to the cell survival network. NCRNA00240, long non-coding RNA with unknown function, was also induced by hyperoxia 3.5 times compared to controls. This indicates, that we found several genes without clear function, what makes functional network analysis more difficult. However, induction of survival related genes and network is relevant and expected. Modification of gene expression seems promising, yet clinically untested, approach in therapy of myocardial infarction (26).
Hyperoxia is known to be associated with excessive formation of reactive oxygen species (ROS), especially after prolonged exposure. Although ROS are commonly related to harmful or physiological effects to the subcellular structures, the beneficial effects of transient ROS formation by mediating the signalling of adaptive defence reaction in mitochondria has been described as well (27, 28). In human microvascular endothelial cells, hyperbaric oxygen induced cytoprotective and angiogenic response against oxidative insults (29), but the changes were minimal following treatment with 100% O2 in the absence of elevated pressure (30). Hyeroxia may also modify the response to different vasoactive stimuli by enhancing vasodilatation in certain circumstances (31).
Similar to short hyperoxic exposure, which causes mild oxidative stress and up-regulation of protective mechanisms (i.e. induces 'readiness' to cope with massive oxidative burden upon ischemia/reperfusion) exposure of H2O2-treated rat myoblasts to nanomolar concentrations of humanin analogue induced activation of catalase and glutathione peroxidase. Further, these cells demonstrated preserved mitochondrial membrane potential, ATP levels and mitochondrial structure, thus resulting in rapid and sustained activation of critical cellular defence systems and attenuation of oxidative stress (32). Similar humanin-induced protection against oxidative stress has been shown in rat retinal cultures (33).
Whether observed changes in the myocardial gene expression after hyperoxic exposure are related to the possible adaption against subsequent oxidative stress, remains speculative. Different gene-expression profiling experiments have led to the discovery of a protective genetic programme activated upon ischemic preconditioning (IPC), and an injury genetic programme activated in response to irreversible ischemic injury, whereas approximately 40% of the genes implicated in IPC are encoded mitochondrial proteins, supporting the importance of mitochondria in IPC mechanism of protection. Genes demonstrating changes in their expression patterns are strongly dependent on the duration and severity of the ischemic insult. All these studies exploring gene expression following different preconditioning stimuli have been recently extensively reviewed (34). In healthy volunteers, remote IPC stimulus suppressed proinflammatory and upregulated anti-inflammatory gene transcription in circulating leukocytes (35). In patients, however, various comorbidities may confound with the tissue response to cardiovascular diseases (36). Hyperoxic preconditioning significantly improves the post-ischaemic functional recovery of rat and mice hearts, reduces the infarct size, and decreases necrotic damage as shown by the reduced release of lactate dehydrogenase. Petrosillo et al. have postulated that in the rat hearts inhibition of mitochondrial permeability transition pore opening and cytochrome c release might by the underlying mechanisms (37). Further, pretreatment with hyperoxia has been shown to reduce apoptotic cell death induced by ischaemic insult increased Bcl-2/Bax ratio (6). Both these aspects, reduced cytochrome c release and reduced apoptosis are related to cytoprotective effects of humanins, as it has been shown that humanin peptides block Bax association with isolated mitochondria, and suppress cytochrome c release in vitro (38).
The study has limitations. First, the patients in the hyperoxia group appeared to be slightly younger. But this is inevitable in a clinical study when age is not considered during randomization. Most probably this minute difference does not result in different gene expression in the human senescent heart, although agerelated differences in the murine hearts after a ischemic preconditioning stimulus have been described (34). Second, the interpretation of genomic expression data is complex, and to determine whether these changes in response to potentially injurious stimuli induce real adaptive/protective pathways, is difficult at the moment. We cannot rule out all confounding factors in our study, because the study group is small and quite rare. However, this limitation is difficult to control in a clinical intervention studies. This study is exploratory and further experiments to study detailed molecular mechanisms and causality are needed. The results of present study should encourage additional studies to analyze the real functional effect and clinical utility of the intervention.
The present study demonstrates that a simple and widely used therapeutic measure ( > 96% oxygen administration for 1 hour) changes gene expression signature in the myocardium of patients with coronary artery disease. Among others, two different humanins were upregulated and survival related genes and network were induced. We propose that upregulation of humanins is a crucial event to afford cytoprotection in the heart and potentially in any other tissue as gene expression was induced in the tissue distant from lungs.
Acknowledgements: This work was supported by the Estonian Science Foundation grants No. 8227, 8736 and 8853.
Conflict of interests: None declared.
REFERENCES
- Tahepold P, Valen G, Starkopf J, Kairane C, Zilmer M, Vaage J. Pretreating rats with hyperoxia attenuates ischemia-reperfusion injury of the heart. Life Sci 2001; 68: 1629-1640.
- Li G, Tokuno S, Tahepold P, Vaage J, Lowbeer C, Valen G. Preconditioning protects the severely atherosclerotic mouse heart. Ann Thorac Surg 2001; 71: 1296-1304.
- Esmaili Dehaj M, Baharvand B, Rasoulian B, et al. Delayed protective effects of hyperoxia against cardiac arrhythmias and infarction in anesthetized rats. J Surg Res 2009; 151: 55-61.
- Tahepold P, Elfstrom P, Eha J, et al. Exposure of rats to hyperoxia enhances relaxation of isolated aortic rings and reduces infarct size of isolated hearts. Acta Physiol Scand 2002; 175: 271-277.
- Pourkhalili K, Hajizadeh S, Tiraihi T, et al. Ischemia and reperfusion-induced arrhythmias: role of hyperoxic preconditioning. J Cardiovasc Med (Hagerstown) 2009; 10: 635-642.
- Foadoddini M, Esmailidehaj M, Mehrani H, et al. Pretreatment with hyperoxia reduces in vivo infarct size and cell death by apoptosis with an early and delayed phase of protection. Eur J Cardiothorac Surg 2011; 39: 233-240.
- Karu I, Loit R, Zilmer K, et al. Pre-treatment with hyperoxia before coronary artery bypass grafting - effects on myocardial injury and inflammatory response. Acta Anaesthesiol Scand 2007; 51: 1305-1313.
- Karu I, Tahepold P, Ruusalepp A, Zilmer K, Zilmer M, Starkopf J. Effects of 60 minutes of hyperoxia followed by normoxia before coronary artery bypass grafting on the inflammatory response profile and myocardial injury. J Negat Results Biomed 2012; 11: 14.
- Bigdeli MR, Rasoulian B, Meratan AA. in vivo normobaric hyperoxic preconditioning induces different degrees of antioxidant enzymes activities in rat brain tissue. Eur J Pharmacol 2009; 611: 22-29.
- Kim CH1, Choi H, Chun YS, Kim GT, Park JW, Kim MS. Hyperbaric oxygenation pretreatment induces catalase and reduces infarct size in ischemic rat myocardium. Pflugers Arch 2001; 442: 519-525.
- Tahepold P, Vaage J, Starkopf J, Valen G. Hyperoxia elicits myocardial protection through a NFκB-dependent mechanism in the rat heart. J Thorac Cardiovasc Surg 2003; 125: 650-660.
- Labruto F, Yang J, Vaage J, Valen G. Role of tumor necrosis factor alpha and its receptor I in preconditioning by hyperoxia. Basic Res Cardiol 2005; 100: 198-207.
- Colantuono G, Tiravanti EA, Di Venosa N, et al. Hyperoxia confers myocardial protection in mechanically ventilated rats through the generation of free radicals and opening of mitochondrial ATP-sensitive potassium channels. Clin Exp Pharmacol Physiol 2008; 35: 64-71.
- Ruusalepp A, Czibik G, Flatebo T, Vaage J, Valen G. Myocardial protection evoked by hyperoxic exposure involves signalling through nitric oxide and mitogen activated protein kinases. Basic Res Cardiol 2007; 102: 318-326.
- Gill R, Kuriakose R, Gertz ZM, Salloum FN, Xi L, Kukreja RC. Remote ischemic preconditioning for myocardial protection: update on mechanisms and clinical relevance. Mol Cell Biochem 2015; 402: 41-49.
- McCarthy DJ, Chen Y, Smyth GK. Differential expression analysis of multifactor RNA-Seq experiments with respect to biological variation. Nucleic Acids Res 2012; 40: 4288-4297.
- Robinson MD, McCarthy DJ, Smyth GK. edgeR: a Bioconductor package for differential expression analysis of digital gene expression data. Bioinformatics 2010; 26: 139-140.
- Storey JD, Tibshirani R. Statistical significance for genomewide studies. Proc Natl Acad Sci USA 2003; 100: 9440-9445.
- Hashimoto Y, Niikura T, Tajima H. A rescue factor abolishing neuronal cell death by a wide spectrum of familial Alzheimer's disease genes and Abeta. Proc Natl Acad Sci USA 2001; 98: 6336-6341.
- Yen K, Lee C, Mehta H, Cohen P. The emerging role of the mitochondrial-derived peptide humanin in stress resistance. J Mol Endocrinol 2013; 50: R11-R19.
- Muzumdar RH, Huffman DM, Calvert J, et al. Acute humanin therapy attenuates myocardial ischemia and reperfusion injury in mice. Arterioscler Thromb Vasc Biol 2010; 30: 1940-1948.
- Oh YK, Bachar AR, Zacharias DG, et al. Humanin preserves endothelial function and prevents atherosclerotic plaque progression in hypercholesterolemic ApoE deficient mice. Atherosclerosis 2011; 219: 65-73.
- Bachar AR, Scheffer L, Schroeder AS, et al. Humanin is expressed in human vascular walls and has a cytoprotective effect against oxidized LDL-induced oxidative stress. Cardiovasc Res 2010; 88: 360-366.
- Zacharias DG, Kim SG Massat AE, et al. Humanin, a cytoprotective peptide, is expressed in carotid atherosclerotic plaques in humans. PLoS One 2012; 7: e31065.
- Widmer RJ, Flammer AJ, Herrmann J, et al. Circulating humanin levels are associated with preserved coronary endothelial function. Am J Physiol Heart Circ Physiol 2013; 304: H393-H397.
- Zimna A, Janeczek A, Rozwadowska N, et al. Biological properties of human skeletal myoblasts genetically modified to simultaneously overexpress the pro-angiogenic factors vascular endothelial growth factor and fibroblast growth factor-4. J Physiol Pharmacol 2014; 65: 193-207.
- Ristow M, Schmeisser S. Extending life span by increasing oxidative stress. Free Radic Biol Med 2011; 51: 327-336.
- Zarse K, Schmeisser S, Groth M. Impaired insulin/IGF1 signaling extends life span by promoting mitochondrial L-proline catabolism to induce a transient ROS signal. Cell Metab 2012; 15: 451-465.
- Godman CA, Joshi R, Giardina C, Perdrizet G, Hightower LE. Hyperbaric oxygen treatment induces antioxidant gene expression. Ann NY Acad Sci 2010; 1197: 178-183.
- Godman CA, Chheda KP, Hightower LE, Perdrizet G, Shin DG, Giardina C. Hyperbaric oxygen induces a cytoprotective and angiogenic response in human microvascular endothelial cells. Cell Stress Chaperones 2010; 15: 431-442.
- van der Sterren S, Kessels I, Perez-Vizcaino F, Cogolludo AL, Villamor E. Prenatal exposure to hyperoxia modifies the thromboxane prostanoid receptor-mediated response to H2O2 in the ductus arteriosus of the chicken embryo. J Physiol Pharmacol 2014; 65: 283-293.
- Klein LE, Cui L, Gong Z, Su K, Muzumdar R. A humanin analog decreases oxidative stress and preserves mitochondrial integrity in cardiac myoblasts. Biochem Biophys Res Commun 2013; 440: 197-203.
- Yang Z, Zhang Q, Ge J, Tan Z. Protective effects of tetramethylpyrazine on rat retinal cell cultures. Neurochem Int 2008; 52: 1176-1187.
- Della-Morte D, Guadagni F, Palmirotta R. Genetics and genomics of ischemic tolerance: focus on cardiac and cerebral ischemic preconditioning. Pharmacogenomics 2012; 13: 1741-1757.
- Konstantinov IE, Arab S, Kharbanda RK, et al. The remote ischemic preconditioning stimulus modifies inflammatory gene expression in humans. Physiol Genomics 2004; 19: 143-150.
- Sharman JE, Johns DP, Marrone J, Walls J, Wood-Baker R, Walters EH. Cardiovascular effects of methacholine-induced airway obstruction in man. J Physiol Pharmacol 2014; 65: 401-407.
- Petrosillo G, Di Venosa N, Moro N, et al. in vivo hyperoxic preconditioning protects against rat-heart ischemia/reperfusion injury by inhibiting mitochondrial permeability transition pore opening and cytochrome c release. Free Radic Biol Med 2011; 50: 477-483.
- Guo B, Zhai D, Cabezas E, et al. Humanin peptide suppresses apoptosis by interfering with Bax activation. Nature 2003; 423: 456-461.
A c c e p t e d : November 2, 2015